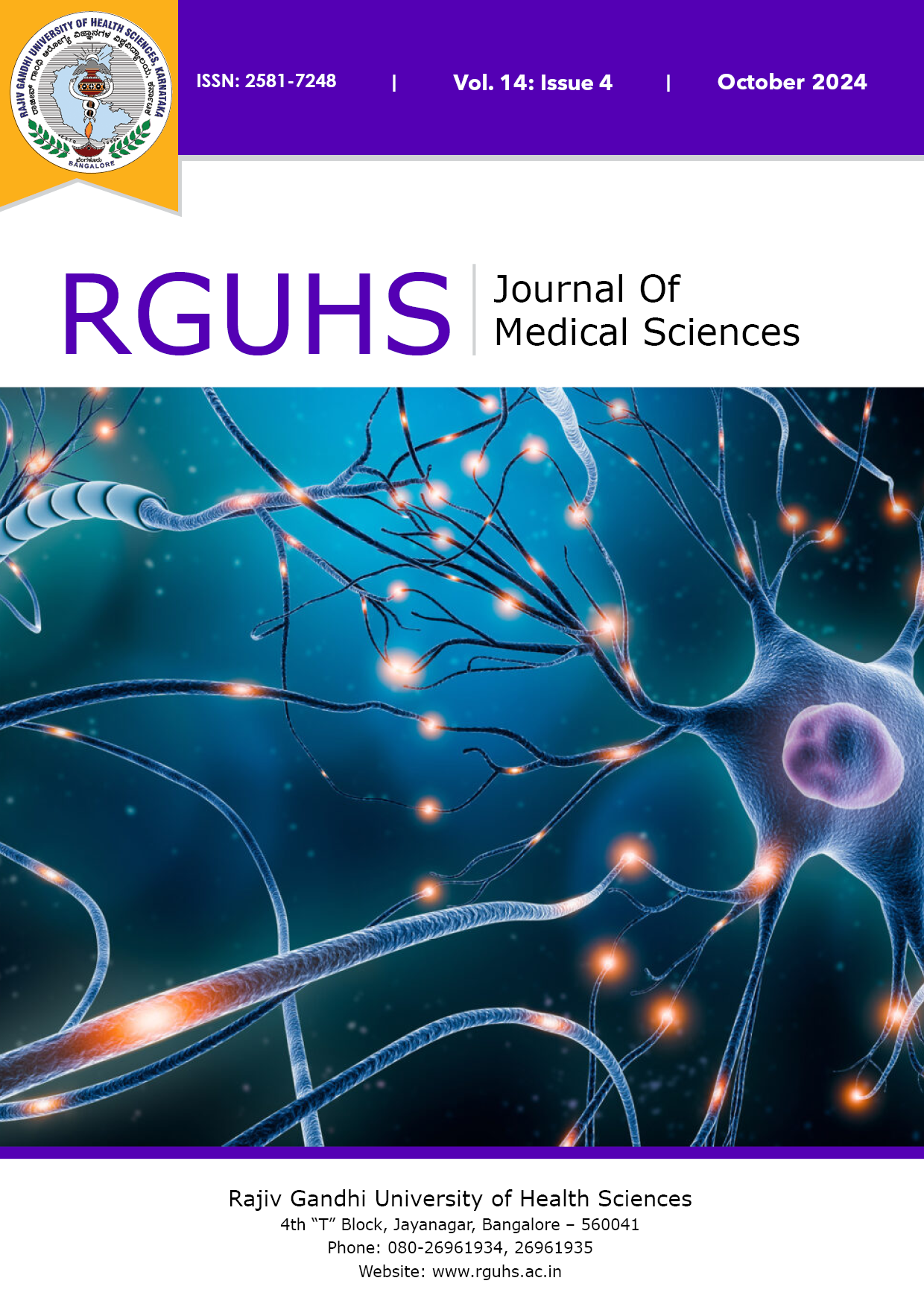
RGUHS Nat. J. Pub. Heal. Sci Vol: 15 Issue: 1 eISSN: pISSN
Dear Authors,
We invite you to watch this comprehensive video guide on the process of submitting your article online. This video will provide you with step-by-step instructions to ensure a smooth and successful submission.
Thank you for your attention and cooperation.
1Department of Orthodontics and Dentofacial Orthopedics, Sri Rajiv Gandhi College of Dental Sciences, Bangalore.
2Department of Periodontics, Sri Rajiv Gandhi College of Dental Sciences, Bangalore.
3Department of Oral and Maxillofacial Pathology, Sri Rajiv Gandhi College of Dental Sciences, Bangalore.
4Department of Orthodontics and Dentofacial Orthopedics, Sri Rajiv Gandhi College of Dental Sciences, Bangalore.
5Department of Prosthodontics, PMNM Dental College and Hospital, Bagalkot, Karnataka.
*Corresponding Author:
Department of Orthodontics and Dentofacial Orthopedics, Sri Rajiv Gandhi College of Dental Sciences, Bangalore., Email: ronykondody55@gmail.com
Abstract
The recent advancement in molecular biology has revolutionized the field of medicine, including various aspects of oral and craniofacial research. These revolutions in genetic engineering enabled the scientific community to understand details regarding gene functions and provide a platform for molecular cures. The latest gene-editing technology in this regard namely CRISPR-Cas system, has opened a new era of versatile genetic manipulations because of its efficiency and ease of operation. Though this technology has enormous applications in different sections of the health care system, this review focuses on explaining the prospect, advances, and insights regarding the future of the CRISPR/Cas system in dental practice.
Keywords
Downloads
-
1FullTextPDF
Article
Introduction
Over the past decade, the scientific development of the new genetic manipulation technology namely CRISPR (Clustered Regularly Interspaced Short Palindromic Repeats) and its related nucleases revolutionised various aspects of genetic engineering which is called genome editing.1 This approach was developed to overcome and address various challenges in the earlier generation gene manipulation technologies. These previous generations worked based on synthetic endonucleases such as zinc finger endonuclease (ZFN) and transcription activator like effector nuclease (TALEN).2 In comparison to prior gene-editing technologies, the third generation CRISPR–Cas9 technology works more accurately and precisely in targeting the genomic sequence with fidelity and minimum off-target effects.3 This procedure begins with the generation of a short piece of guide RNA that in turn binds to Cas9, making base pairing that targets DNA.4 The Cas9 nuclease then slices DNA at a specific area, breaking both DNA strands. The repair mechanism of the cell then inserts or removes, or replaces existing DNA with tailored sequences. This procedure is continually being modified and offers a wide range of potential applications in the treatment of various genetic conditions.5-7
Currently, genomic dentistry serves two primary purposes: preventive and predictive.8 Due to the severity and morbidity associated with various disorders and developmental anomalies, researchers are now more focused to investigate gene activities on a genome-scale that will in turn help in the management of these conditions. Furthermore, genome editing has substantial translational and therapeutic promise.1 Various studies have been conducted in this regard about human genome variation for numerous oral and craniofacial conditions.8
Therefore this review focused to provide a comprehensive summary of major genome-editing nucleases, along with various aspects of the CRISPR/Cas9 system in dentistry.
Working Mechanism and Characteristics of CRISPR/ Cas Gene
CRISPR/Cas molecular system is an adaptive response that is immunologically mediated in bacteria and archaea that uses endonuclease to target viral DNA. Over the years, development occurred in various aspects of CRISPR/Cas gene technology.9 (Table 1) This system employs RNA-DNA base-pairing processes that consist of three steps: acquisition, expression, and interference.
The combination of Cas1 and Cas2 proteins completes the first step. In this case, the protein complex identifies the protospacer and protospacer adjacent motif (PAM) in foreign nucleic acids, which is captured and incorporated as new CRISPR spacers into a CRISPR array separated by repeat sequences, resulting in an “immune memory” of invading genetic material.10
As part of the genomic mechanism, three kinds of CRISPR/Cas systems are accessible.11 The type II CRISPR/Cas approach is the most prevalent and it consists of an endonuclease (Cas9), a CRISPR RNA (crRNA), and also a transactivating crRNA. Both crRNA and tracrRNA molecules join to create a guide RNA which in turn may be replaced by a synthetic fused chimeric single gRNA that will help in gene editing.12
The synthetic gRNA consists of 20 base-pair which are followed by a short DNA known as the PAM that is designed to target the DNA.13,14 When sgRNA and Cas9 nuclease are produced in the cell, they create a ribonucleoprotein (RNP) complex that is steered to a target DNA region. Cas9 accurately cleaves the DNA to create a double-strand break (DSB), resulting in blunt ends, and sgRNA attaches to the target sequence.
DNA repair pathway is divided into:15
a. Non-homologous end joining (NHEJ) pathway: It is a quick-fix mechanism; however, it is a DSB repair route that is prone to errors. By detecting DSBs, this pathway cleans the DNA ends, recruits kinases and ligases, and finally ligates the damaged DNA ends. It is subdivided into two subclasses namely, canonical and alternative. It frequently results in precise end joining or deletions, whereas NHEJ produces bigger deletions.
b. Homology-directed repair (HDR) pathway: This technique promotes more accurate DNA repairs through a double-stranded donor template. It may either restore the original DNA contents or result in exact replacements such as point mutations or gene insertions, depending on the donor template sequences. C-NHEJ inhibition increases the frequency of alt-NHEJ and HDR events. These DNA repair processes set the groundwork for CRISPR/Cas9 biological applications.
Applications of CRISPR/Cas9 Genomic Technology
CRISPR/Cas9 technology has gained substantial importance recently in the field of research and is a potent therapeutic tool for treating disorders related to genome abnormalities by eliminating the mutations and replacing them with ideal DNA sequences.16
In cases of cancer immunotherapy, CRISPR/Cas9 works by enhancing the synthesis of immune cells, like chimeric antigen receptor T (CAR-T) cells and by deletion of programmed cell death protein 1 knockout.17 CAR has an intracellular chimeric signalling domain capable of stimulating T cells and an external single-chain variable segment capable of recognising tumour antigens, perhaps providing beneficial outcomes in instances of leukaemia and lymphomas.18-20
CRISPR/Cas9 System and Dentistry
Gene therapy in dentistry is still in its initial stages which is used to manage various conditions like squamous cell carcinoma, tooth and bone regeneration, salivary gland illness, and also as a part of orthodontic treatment.21 CRISPR/Cas9 genome editing tools, in particular, have considerably aided biological research. Precision based genome editing techniques will allow us to acquire a better understanding of the genes implicated in mouth disorders and craniofacial abnormalities.1
Genetic manipulation of orthodontic tooth movement
Gene therapy trials in orthodontics are still at cell culture or animal experiment levels.22 Past gene therapy in the field of orthodontic procedures sought to study the possibilities of accelerating tooth movement or decreasing root resorption by altering osteoclast differentiation factors. The first gene therapy in orthodontics intended to introduce the OPG gene into periodontal tissue to suppress osteoclast activity and limit tooth movement.23,24 The gene transfer method was used in rats for 21 days of tooth movement utilising a hemagglutinating virus of Japan envelope vector containing mouse OPG messenger RNA (mRNA). The results demonstrated that local OPG gene transfer reduced the number of osteoclasts and tooth movement in rats in the experimental group by 50% as compared to rats in the control group. The effect of OPG gene transfer was limited and did not influence the animals’ tibial bone mineral density.25 With advanced technology, the manufacturer is now able to produce human recombinant proteins for medicine including therapeutic applications. However, once injected into the human body, these proteins have a limited half-life and may not reach a therapeutic level.26
CRISPR/Cas9 and periodontal diseases
Porphyromonas gingivalis is considered the keystone pathogen associated with periodontal disease. Up to 95% of the clinical strains of P. gingivalis bear the CRISPR arrays and this genetic immune system within the microbe may be associated with regulating the pathogenesis of chronic periodontitis.27
CRISPR–Cas9 can be helpful to create knockout (CRISPR KO) models as a screening method to develop cellular pathways in the pathogenesis of periodontitis. These systems, CRISPRa, CRISPRi, and Cas13 can modify the transcriptome and gene expression of certain genes without changing the sequence of the genome which may result in periodontitis.3
Cas3 can target the periodontal biofilm and eliminate periodontal pathogens. Future research of the CRISPR systems against pathologic biofilms can facilitate the development of newer therapeutic regimens to eliminate periodontal disease.
The CRISPR/Cas9 system can also influence tooth and palate development by targeting the C-terminal domain of the Msx1 gene. In a study by Mitsui SN et al., the investigators targeted Msx1 with a CRISPR/Cas system that showed agenesis of lower incisors with or without cleft palate.28 This technique also helps in the management of temporomandibular disorders by targeting genome modification by RNA guided transcriptional activators and repressors targeted to genes that are related to pain pathways.29
Ethical Issues of CRISPR/Cas9 Technology
Gene therapy appears to be a viable therapeutic option for a variety of disorders. This methodology is still working as an alternate means of treating deformities or diseases that traditional procedures cannot reach. Although several clinical trials have demonstrated success, the approach is currently being studied for its safety concerning any hereditary or systemic consequences.
The most significant restriction of CRISPR/Cas9 technology is off-target effects, resulting in unexpected genetic alterations. Schaefer et al., 30 discovered increased cases of harmful mutations in two CRISPR/Cas9- modified mice, leading to rise in concerns regarding the use. Although these mutations are uncommon, they must not be overlooked. If a gene is changed other than the target, an undesirable clinical consequence may emerge; consequently, careful optimization and trials are required to limit this risk.31
Future Perspectives and Scope for Research
With the advancement in biomedical engineering, genetic treatment is no more considered science fiction. Over the last decade, many procedures have been introduced with gene targeting with endogenous microRNA (miRNA) for targeted gene delivery. These miRNAs are short, non-coding RNA sequences, controlling gene expression by binding to the target region in the matching mRNAs.32
Many additional CRISPR-associated enzymes are being researched in addition to Cas9. Cpf1 enzyme mimics Cas9 in DNA slicing and possesses a variety of deactivated versions which can be employed to explore transcriptional effects.33 Csm6 enzyme functions similarly to Cas13 ribonuclease.34 The quest for new CRISPR-associated nucleases will be substantially aided by microbial whole-genome sequencing and improved structural biology.
Nevertheless, to change nucleotide bases at the genome level, various techniques use enzymes to act directly. Also, transfer RNA adenosine deaminase editors coupled with nCas9 can change an A-T bp to a G-C pair at the specified site in the genome without causing DSB.35 It is still essential to build fused enzymes to catalyse additional conversions, but this discovery is a significant step towards the next generation of genome-editing technology that does not need DSBs.
Conclusion
With rapid progress in genetic engineering in health care and scientific research, the new system of CRISPR Gene-editing technology has provided new avenues for the management of various genetic conditions. Also, with the advent of this genetic manipulation system, our understanding of various disease pathogenesis will improve and also create an important tool in clinical scenarios to alter the host cells and genomes, thus changing the course of disease severity.
Financial assistance
Nil
Conflicts of Interest
Nil
Supporting File
References
- Yu N, Yang J, Mishina Y, Giannobile WV. Genome editing: A new horizon for oral and craniofacial research. J Dent Res 2019;98(1):36-45.
- Doudna JA, Charpentier E. Genome editing. The new frontier of genome engineering with CRISPRCas9. Sci 2014;346:1258096.
- Barbour A, Glogauer J, Grinfeld L, Ostadsharif Memar R, Fine N, Tenenbaum H, et al. The role of CRISPR-Cas in advancing precision periodontics. J Periodontal Res 2021;56(3):454-461.
- Hryhorowicz M, Lipianki D, Zeyland J, Slomski R. CRISPR/Cas9 Immune System as a Tool for Genome Engineering. Arch Immunol Ther Ex 2017;65(3):233-240.
- Beaudet AL, Meng L. Gene-targeting pharmaceuticals for single-gene disorders. Hum Mol Genet 2016;25(R1):R18-R26.
- Morishige S, Mizuno S, Ozawa H, Nakamura T, Mazahery A, Nomura K, et al. CRISPR/Cas9- mediated gene correction in hemophilia B patient-derived iPSCs. Int J Hematol 2020;111(2):225-233.
- Schwank G, Koo BK, Sasselli V, Dekkers JF, Heo I, Demircan T, et al. Functional repair of CFTR by CRISPR/Cas9 in intestinal stem cell organoids of cystic fibrosis patients. Cell Stem Cell 2013;13(6):653-658.
- Chavez-Granados PA, Manisekaran R, Acosta-Torres LS, Garcia-Contreras R. CRISPR/Cas geneediting technology and its advances in dentistry. Biochimie 2022;194:96-107.
- Wang D, Wang XW, Peng XC, Xiang Y, Song SB, Wang YY, et al. CRISPR/Cas9 genome editing technology significantly accelerated herpes simplex virus research. Cancer Gene Ther 2018;25(5-6):93- 105.
- Pourcel C, Salvignol G, Vergnaud G. CRISPR elements in Yersinia pestis acquire new repeats by preferential uptake of bacteriophage DNA, and provide additional tools for evolutionary studies. Microbio 2005;151:653–63.
- Jinek M, Chylinski K, Fonfara I, Hauer M, Doudna JA, Charpentier E. A programmable dual-RNAguided DNA endonuclease in adaptive bacterial immunity. Sci 2012;337:816–821.
- Martinez-Lage M, Puig-Serra P, Menendez P, Torres-Ruiz R, Rodriguez-Perales S. CRISPR/Cas9 for cancer therapy: hopes and challenges. Biomed 2018;6(4):105.
- Chen S, Sun H, Miao K, Deng CX. CRISPR-Cas9: from genome editing to cancer research. Int J Biol Sci 2016;12(12):1427–1436.
- Betermier M, Bertrand P, Lopez BS. Is non-homologous end-joining really an inherently errorprone process? PLoS Genet 2014;10(1):e1004086.
- Hu Z, Shi Z, Guo X, Jiang B, Wang G, Luo D, et al. Ligase IV inhibitor SCR7 enhances gene editing directed by CRISPR-Cas9 and ssODN in human cancer cells. Cell Biosci 2018;8:12.
- Zhan, T., Rindtorff N, Betge J, Ebert MP, Boutros M. CRISPR/Cas9 for cancer research and therapy. Semin Cancer Biol 2019;55:106–119.
- Xia AL, He QF, Wang JC, Zhu J, Sha YQ, Sun B, et al. Applications and advances of CRISPR-Cas9 in cancer immunotherapy. J Med Genet 2019;56(1):4- 9.
- Neelapu SS, Tummala S, Kebriaei P, Wierda W, Gutierrez C, Locke FL, et al. Chimeric antigen receptor T-cell therapy - assessment and management of toxicities. Nat Rev Clin Oncol 2018;15:47–62.
- Maus MV, Grupp SA, Porter DL, June CH. Antibody-modified T cells: CARs take the front seat for hematologic malignancies. Blood 2014;123:2625– 35.
- Brudno JN, Kochenderfer JN. Chimeric antigen receptor T-cell therapies for lymphoma. Nat Rev Clin Oncol 2018;15:31–46.
- Coppari R, Bjørbæk C. Leptin revisited: its mechanism of action and potential for treating diabetes. Nat Rev Drug Disco 2012;11:692–708.
- Siddique N, Raza H, Ahmed S, Khurshid Z, Zafar MS. Gene therapy: A paradigm shift in dentistry. Genes (Basel) 2016;7(11):5-12.
- Abraham P, Maliekal TT. Single cell biology beyond the era of antibodies: Relevance, challenges, and promises in biomedical research. Cell Mol Life Sci 2017;74(7):1177-1189.
- Kanzaki H, Chiba M, Takahashi I, Haruyama N, Nishimura M, Mitani H. Local OPG gene transfer to periodontal tissue inhibits orthodontic tooth movement. J Dent Res 2004;83(12):920-925.
- Zhao N, Liu Y, Kanzaki H, Liang W, Ni J, Lin J. Effects of local osteoprotegerin gene transfection on orthodontic root resorption during retention: An in vivo micro-CT analysis. Orthod Craniofac Res 2012;15(1):10-20.
- Iglesias-Linares A, Moreno-Fernandez AM, Yanez-Vico R, Mendoza-Mendoza A, Gonzalez-Moles M, Solano-Reina E. The use of gene therapy vs. corticotomy surgery in accelerating orthodontic tooth movement. Orthod Craniofac Res 2011;14(3):138-148.
- Makarov SS, Olsen JC, Johnston WN, Anderle SK, Brown RR, Baldwin AS Jr, et al. Suppression of experimental arthritis by gene transfer of interleukin 1 receptor antagonist cDNA. Proceedings of the National Academy of Sciences of the United States of America. 1996;93(1):402-406.
- Chen T, Olsen I. Porphyromonas gingivalis and its CRISPR-Cas system. J Oral Microbio 2019;11(1):1638196.
- Mitsui SN, Yasue A, Masuda K, Naruto T, Minegishi Y, Noji S, et al. Novel human mutation and CRISPR/ cas genome-edited mice reveal the importance of C-terminal domain of MSX1 in tooth and palate development. Sci Rep 2016;6:38398.
- Munzenmaier DH, Wilentz J, Cowley AW Jr. Genetic, epigenetic, and mechanistic studies of temporomandibular disorders and overlapping pain conditions. Mol Pain 2014;10:72.
- Schaefer KA, Wu WH, Colgan DF, Tsang SH, Bassuk AG, Mahajan VB. Unexpected mutations after CRISPR-Cas9 editing in vivo. Nat Methods 2017;14(6):547–548.
- Plaza Reyes A, Lanner F. Towards a CRISPR view of early human development: applications, limitations and ethical concerns of genome editing in human embryos. Development 2017;144(1):3-7.
- Li B, Zeng C, Dong Y. Design and assessment of engineered CRISPRCpf1 and its use for genome editing. Nat Protoc 2018;13(5):899–914.
- Niewoehner O, Garcia-Doval C, Rostol JT, Berk C, Schwede F, Bigler L, et al. Type III CRISPRcas systems produce cyclic oligoadenylate second messengers. Nature 2017;548(7669):543–548.
- Gaudelli NM, Komor AC, Rees HA, Packer MS, Badran AH, Bryson DI, et al. Programmable base editing of A•T to G•C in genomic DNA without DNA cleavage. Nature 2017;551(7681):464–471