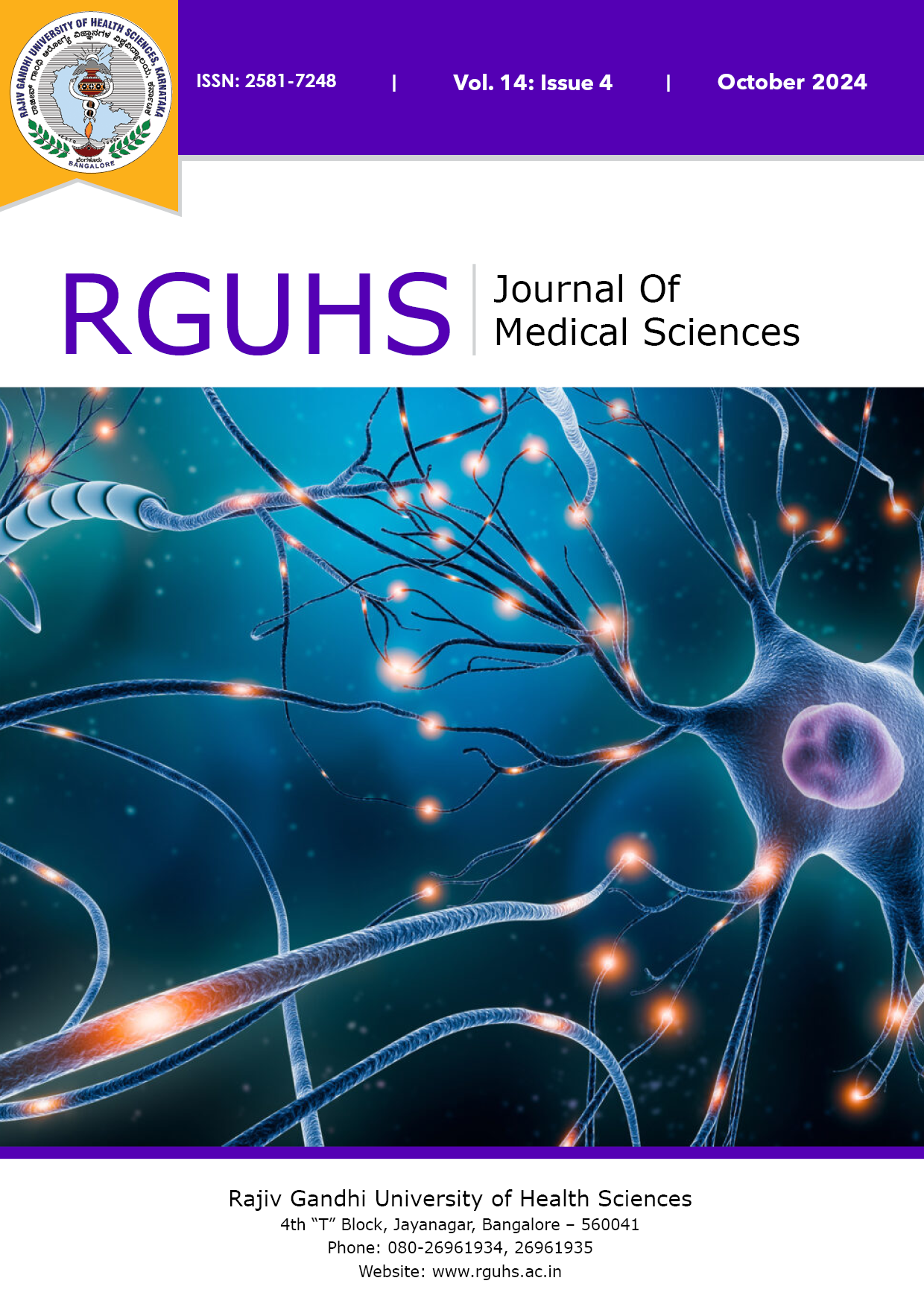
Abbreviation: RJMS Vol: 15 Issue: 1 eISSN: 2581-7248 pISSN 2231-1947
Dear Authors,
We invite you to watch this comprehensive video guide on the process of submitting your article online. This video will provide you with step-by-step instructions to ensure a smooth and successful submission.
Thank you for your attention and cooperation.
Shankar P S
Editor-in-Chief, RJMS, Emeritus Professor of Medicine: RGUHS

Abstract
None
Keywords
Downloads
-
1FullTextPDF
Article
Alzheimer’s disease (AD), the most common cause of dementia, is a progressive and fatal neurodegenerative disorder. It is characterized pathologically by atrophy of the cerebral cortex and hippocampus, with intraneuronal neurofibrillary tangles containing abnormally phosphorylated tau protein, extracellular amyloid plaques, and neuronal cell death. It is characterized clinically by gradual impairment of memory.1 As the disease progresses, the patient becomes impaired in both cognitive and functional capacities. The loss of intellectual abilities is severe to interfere with social and occupational functioning.
The sensory experiences received by the human brain are processed and stored as memory. This information is recalled in an integrated manner at an appropriate time. Memory fades in AD and it is often compared to the erasure of a computer hard disk. Initially, it involves failure to recall the recent events though the person can recollect the events that had taken place long ago. As the illness progresses, the old memory also disappears, and ultimately the patient fails to recognize the near and dear ones. This memory-destroying illness is associated with a loss of lifetime memories that make up the identity of the person.
The disease is associated with the destruction of more than 100 billion neurons and their associated 100 trillion connections. There is a progressive loss of cortical neurons and formation of amyloid plaques, intraneuronal neurofibrillary tangles, and accumulation of a beta-amyloid in the arterial walls of cerebral blood vessels (amyloid angiopathy). Beta-amyloid is the major component of the plaques, whereas hyperphosphorylated tau protein is the major constituent of the neurofibrillary tangles. The pathological process of atrophy begins in the hippocampus and spreads to diffuse areas of the temporal, parietal, and frontal lobes of the cerebral cortex. There is a symmetric enlargement of the third and fourth ventricles. The loss of neurons, especially in the nucleus basalis causes a relative deficiency of acetylcholine resulting in different clinical manifestations.
The neurotransmitter, acetylcholine is necessary for clear thinking. It gets destroyed by the enzyme acetylcholinesterase (ChE). Since acetylcholine deficiency has been observed in AD, ChE inhibitors have been used to block the action of ChE to increase the cerebral concentration of acetylcholine essential for synaptic transmission. Donepezil, rivastigmine, and galantamine (ChE inhibitors) facilitate an increase in the level of acetylcholine.2 These agents show improvement in global function and reduce cognitive disturbances. There is a reduction in behavioral disturbances and temporary stabilization of activities of daily living.3 As the destruction of the neurons proceeds relentlessly the medications become ineffective after some time.
The symptoms of AD are thought to be due to persistent activation of the central nervous system’s N-methyl-Daspartate (NMDA) receptors by glutamate. Glutamate acts as the main excitatory neurotransmitter. Memantine, an NMDA receptor antagonist acts either by interfering with glutamatergic excitotoxicity or by providing symptomatic improvement through effects on functions of hippocampal neurons.4 Though it slows the cognitive decline in mild-to-moderate AD, its effects do not last long.
Efforts are made to find treatment to slow or halt AD following a better understanding of the molecular events that appear to trigger it. It has kindled the hope of effectively slowing or stopping the gradual loss of neurons in the brain, and ultimately stopping the progression of the disease. Many drugs are in various stages of clinical trials and there are some promising preliminary results.5
Amyloid plaques and tangles: A cascade of events pertaining to amyloid lie in the development of AD.6 The amyloid cascade hypothesis is based on the fact that plaques and tangles of proteins in the cerebral cortex and limbic system deleteriously affect the higher functions of the brain. The plaques are deposited outside the neurons and are composed of a small protein called amyloid beta. The tangles are found inside neurons, and their branching axons and dendrites. They are made up of filaments of proteins called tau. The plaques and tangles are responsible for the degeneration of the neurons. Amyloid-beta triggers the disruption and death of the neurons.
This hypothesis has led to the development of drugs to inhibit the production of amyloid beta and tau and stop the harmful effects of them on neurons.
Amyloid beta is a short peptide. It is derived from the amyloid precursor protein (APP). One part of the protein is inside the cells and the other part sticks out of the cellular membrane. Two protease enzymes- beta-secretase and gamma-secretase can carve out amyloid beta from APP. This is a normal process occurring in all cells of the body.
In AD, there is an excess accumulation of amyloid beta. Initially, beta-secretase cuts APP found outside the cellular membrane with the help of aspartic acids. Then the presenilin protein, a component of the gamma-secretase enzyme cuts the remaining portion of APP found inside the membrane and releases amyloid beta into the aqueous environment outside the membrane, and gets attached as small soluble assemblies (plaques). They are toxic to the neurons. Experimentally it has been shown that high concentrations of amyloid beta molecules in a test tube can assemble into fibrillary structures similar to those found in the plaques of AD. They are toxic to neurons cultured in Petri dishes.
The step-wise process of oxidation and lipid peroxidation of cell membranes, glutamatergic excitotoxicity, betaamyloid aggregation, inflammation, and tau hyper phosphorylation, cause neurotoxicity, neuronal cell death, and neurotransmitter deficit.
Neuritic plaques have a central core of insoluble deposit of amyloid beta-peptide surrounded by astrocytes, microglia, and dystrophic neuritis consisting of paired helical filaments.7 Neurofibrillary tangles are made up of paired helical filaments of abnormally filled and phosphorylated tau protein in the neuron and its dendrites. More tau tangles are seen in the brain as the disease advances. There is also a reduction in the synaptic density, loss of neurons, and degeneration in hippocampal neurons. There is a specific degeneration of neurons concerned with the maintenance of specific transmitter cysteines and results in deficits of acetylcholine, norepinephrine, and serotonin.8 Though plaque formation arrests, plaque formation of tangles continue. It correlates with the progress and severity of dementia.
Genetic predisposition: Members of the families having a high risk of AD at a relatively young age carry rare genetic mutations that encode APP specifically affecting the areas of the protein in and around the amyloid beta region. Genetic predisposition appears to be inherited as an autosomal dominant trait with relatively complete penetrance. Four different genes have been identified to be involved in the heritable form of the disease. The presence of the apolipoprotein E4 allele found on the long arm of chromosome 19 increases the likelihood of the development of AD.9 Apolipoprotein E4 genotype appears to enhance amyloid beta peptide aggregation or decrease its cleavage. This makes them susceptible to developing the disease at a relatively young age. It has been shown that people with Down’s syndrome (trisomy 21) exhibit a much higher incidence of AD in middle age. This is due to the fact chromosome 21 contains the APP gene. There is an increased production of amyloid beta from birth, and consequently, an increased amyloid deposit from a young age.
Mutations in two related genes called presenilin 1 and 2 lead to the occurrence of the severe form of AD early in life. The mutations increase the amount of amyloid beta that is prone to clumping. Mutations of the presenilin-1 gene located on chromosome 14 may lead to an early onset autosomal dominant AD.10 Rarely the mutations of the presenilin-2 gene on chromosome 1 may cause autosomal dominant AD. It occurs with an early onset of the disease and a shorter, more rapidly progressive course. The proteins encoded by the presenilin genes are a part of the gamma-secretase enzyme that helps in the manufacture of the harmful peptides
Protease inhibitors: It is not clear how amyloid beta destroys the neurons. Aggregates of amyloid beta found outside the neuron can initiate a cascade of events that can bring about an alteration of the tau protein inside the cell. These aggregates change the kinases that add phosphates to proteins. There is a likelihood of the addition of an excess amount of phosphates to tau, resulting in the formation of twisted filaments. The altered tau proteins are likely to act deleteriously by disrupting the microtubules carrying proteins along the axons and dendrites, and kill neurons. Thus amyloid beta plays a pivotal role in the initiation of AD. Drugs are being produced that target the proteases (protease inhibitors) that produce amyloid beta, and inhibit their activity.
The proteases use aspartic acids to catalyze protein cutting reactions. Small-sized beta-secretase inhibitors are yet to be developed that can effectively pass through the bloodbrain barrier. Gamma-secretase is the other enzyme involved in the formation of amyloid beta by cutting the remaining portion of APP inside the cell following the cleavage by beta-secretase. Studies in mice have shown that deletion of the presenilin-1 gene genetically decreases the cutting of APP by gamma-secretase. It has been proved that the protein encoded by the gene is essential for the function of the enzyme. Inhibitors of aspartyl proteases could block gamma-secretase cleavage of APP in the cells. Gamma-secretase also contains a pair of aspartic acids as in beta-secretase and is essential for catalyzing the proteincutting reaction.11 Presenilin protein acts like an unusual aspartyl protease in the cell membranes. The inhibitors of gamma-secretase are relatively small molecules that can penetrate the blood-brain barrier.
Inhibitors of aspartyl proteases could block gammasecretase cleavage of APP in cells.. Two aspartic acids in presenilin lie within the membrane. They are essential in the gamma-secretase cleavage to produce amyloid beta. Inhibitors of gamma-secretase bind directly to presenilin. The gamma-secretase enzyme plays an important role in the maintenance of undifferentiated precursor cells in different parts of the body. They cut a cell surface protein called notch receptor. High doses of gamma-secretase inhibitors cause toxic effects in mice by disrupting the amyloid notch signal. Molecules have been identified that modulate gamma secretes so that amyloid beta production is blocked without affecting the cleavage of the notch.11 Attempts have been made to produce inhibitors that can curtail the creation of amyloid beta or create a shorter peptide that does not clump easily. Such a preparation called, Flurizan has shown promising results
Immunization: The second strategy is to clear the brain of toxic assemblies of amyloid beta after its production by active immunization. It involves recruiting the patient’s immune system to attack amyloid beta. Injection of amyloid beta into the genetically engineered mice to develop amyloid plaques stimulated an immune response that prevented the plaques from forming in the brain of young mice and cleared plaques already present in the older mice.11
The mice produced antibodies that recognize amyloid beta and the antibodies made the microglia in the brain attack aggregates of the peptide. It improved learning and memory. However, studies in humans have led to the development of encephalitis probably through the action of T-cells. But immunization produced antibodies against amyloid beta and there was some improvement in memory and concentration. Passive immunization by injecting the antibodies into patients aims to clear the peptides. These antibodies produced in mouse cells and genetically engineered to prevent rejection in humans are unlikely to evoke the occurrence of encephalitis as they do not trigger the T-cell response in the brain. The procedure was able to remove amyloid beta from the brain.. Immunization with selected parts of amyloid beta instead of an entire peptide can stimulate the antibody-producing B-cells of the immune system without triggering T-cells involved in the occurrence of encephalitis.
Non-immunological strategy: Non-immunological strategy to stop the aggregation of amyloid beta compounds has been attempted. The compounds interact directly with amyloid beta to keep the peptide dissolved in the fluid outside brain neurons, thereby preventing the formation of harmful clumps. Alzhemed, a small molecule mimicking heparin, binds to amyloid beta and reduces peptide aggregation, and shows some improvement in the cognitive functions of patients with mild AD.
Targetting Tau: The tau filaments cause neuronal tangles, and they are a promising target to prevent the degeneration of neurons. Inhibitors could block the kinases that place an excessive amount of phosphates onto tau, which is an essential step in filament formation. No success was seen in the production of such a drug. It is hoped such drugs might work synergistically with those targeting amyloid beta.
Reduction in production of APP: Cholesterol lowering agents (statins) used to decrease the risk of heart disease could become a treatment for AD. Epidemiologic studies have shown that people taking statins have a lower risk of acquiring AD. By lowering cholesterol these drugs may reduce the production of APP or affect the creation of amyloid beta by inhibiting the activity of the responsible secretases. Attempts are being made to prevent AD by using statins.
Cell-based therapy: Cell therapy is another approach for treatment. The gene encoding a large protein such as nerve growth factor (NGF) was inserted into the skin biopsies obtained from patients with mild AD. Such genetically modified cells were implanted surgically into the forebrain of the patients, as implanted cells produce and secrete NGF, thus preventing the loss of acetylcholineproducing neurons and improving memory. The treatment was associated with slowing cognitive decline.
Targetting the amyloid cascade has attracted novel neurotherapeutics for AD. It has led to an approach to prevent amyloid beta accumulation in the brain by developing beta-secretase inhibitors and designing direct inhibitors of amyloid beta aggregation.12 The amyloid cascade hypothesis following the identification of APP has been established and the recognition of amyloid beta to be the principal protein component of senile plaques. The amyloid cascade is closely involved in AD and amyloid beta is responsible for neurodegenerative processes in the disease.13
The medical fraternity is eagerly looking forward to a breakthrough in the research to provide a drug that could effectively slow or stop the gradual loss of neurons. The treatment targeting amyloid beta may halt the occurrence or retard the progress of AD.
Supporting File
References
1. Cummings JL, Cole G. Alzheimer Disease. JAMA 2002; 287: 235-2338
2. Doody RS, Stevens JC, Buck C, et al. Practice parameter: Management of dementia (an evidencebased review): report of the quality standards subcommittee of the American Academy of Neurology. Neurology 2001; 293: 1446-1447
3. Cummings R. Cholinesterase inhibitors: a new class of psychotropic agents. Am J Psychiatr 2000; 157: 6-15
4. Tariot PN, Farlow MR, Grossberg GT, Graham SM, McDonald S, Gergel I. Memantine treatment in patients with moderate to severe Alzheimer disease already receiving donepezil: a randomized controlled trial. JAMA 2004; 291: 317-24
5. Wolfe MS. Therapeutic strategies for Alzheimer’s disease. Nat Rev Drug Discov 2002; 1: 859-866.
6. Selkos DJ. Presenilins, B-amyloid precursor protein and the molecular basis of Alzheimer’s disease. Clin Neurol Res 2001; 1: 91-103
7. Cummings R, Vinters HV, Cole GM, Khachaturian ZS. Alzheimer’s disease. Neurology 1998; 51: 53-57
8. Palmer AM, Stratmann GC, Proctor AW, Bowen DM. Possible neurotransmitter basis of behavioral changes in Alzheimer’s disease. Ann Neurol 1988; 23: 61020
9. Seshadri S, Drachman DA, Lippa CF. Apolipoprotein E4 allele and the lifetime risk of Alzheimer’s disease. Arch Neurol 1995; 52: 1074-1079
10. Scheelenberg GD, Bird TD, Weijsman EM, et al. Genetic linkage evidence for a familial Alzheimer’s disease locus on chromosome 14. Science 1992; 258: 668-671
11. Wolfe MS. Shutting down Alzheimer’s. Sci Amer India 2006; 1: 60-67
12. Mohammed T, Shakeri A, Rao PPN. Amyloid cascade in Alzheimer’s disease: Recent advances in medicnal chemistry. Eur Jour Med Chem 2016: 113: 258-272
13. Castellani I, Plascencia-Villa G, Perry G. The amyloid cascade and Alzheimer’s disease therapeutics: theory versus observation. Lab Invest 2019 99:958–970