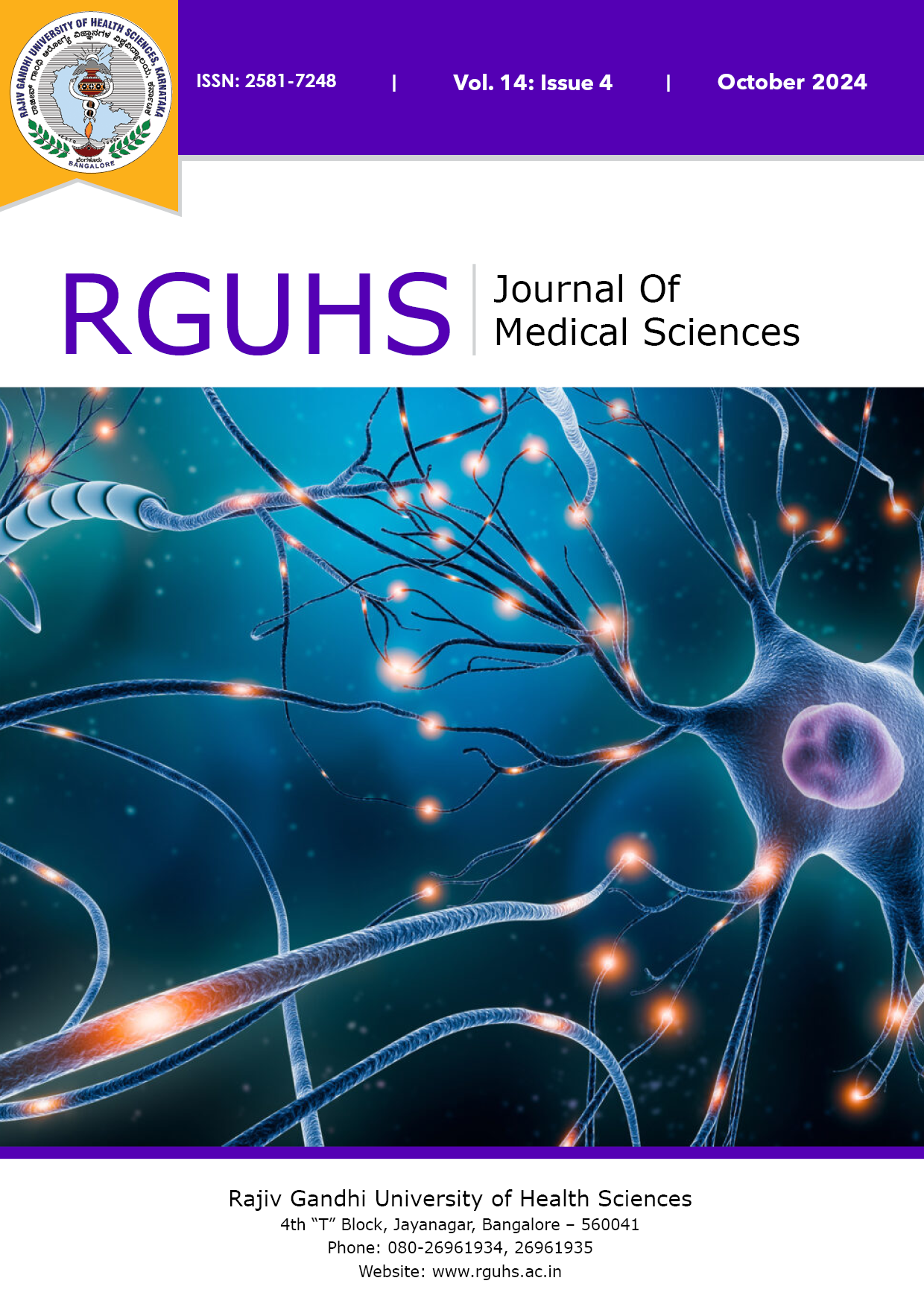
RGUHS Nat. J. Pub. Heal. Sci Vol: 15 Issue: 1 eISSN: pISSN
Dear Authors,
We invite you to watch this comprehensive video guide on the process of submitting your article online. This video will provide you with step-by-step instructions to ensure a smooth and successful submission.
Thank you for your attention and cooperation.
Priyanka A1 , Debalina Sen2 , Wilma Delphine Silvia CR3*
1,2,3Department of Biochemistry, Sri Atal Bihari Vajpayee Medical College, Research Institute, Bangalore.
*Corresponding author:
Dr. Wilma Delphine Silvia CR, Professor and Head, Department of Biochemistry, Sri Atal Bihari Vajpayee Medical College and Research Institute, Affiliated to RGUHS, Bengaluru - 560001. Email: bowringbiochem@gmail.com
Received date: December 12, 2020; Accepted date: February 21, 2021; Published date: March 31, 2021

Abstract
The whole world is reeling under the coronavirus disease-2019 (COVID-19) pandemic. It is caused by the novel coronavirus severe acute respiratory syndrome coronavirus-2 (SARS-CoV-2). The diagnosis of COVID-19 is based on clinical manifestations, history of exposure, positive findings on chest computerized tomography (CT), and laboratory tests. It has been reported that increased release of pro-inflammatory mediators due to an overactive immune system plays a pivotal role in the pathogenesis of COVID-19. This review provides an insight into the different types of coronavirus strains and inflammatory profile involved in COVID-19 with special emphasis on biomarkers such as procalcitonin, lactate dehydrogenase (LDH), ferritin, D-dimer, and cytokines.
Keywords
Downloads
-
1FullTextPDF
Article
Introduction
Inflammation is the body’s internal mechanism of responding to any infection, surgery, trauma, burns, cancer, etc. During inflammation, certain types of proteins are released into the bloodstream. If there is at least 25% increase or decrease in their concentration, these proteins are considered as systemic inflammatory markers. C-reactive protein (CRP), erythrocyte sedimentation rate (ESR), and plasma viscosity (PV) are commonly employed in the diagnosis and monitoring of inflammatory conditions, including infections, autoimmune conditions, and cancers. Testing for the inflammatory markers, especially CRP, has increased over the last 15 years.1
There are several inflammatory markers; however, only few markers called acute phase reactants, such as CRP, ESR, and procalcitonin (PCT), are commonly used in clinical practice. Although they are nonspecific and cannot be used for diagnosing any particular disorder, they aid in finding some common inflammatory stages during the diagnosis. PCT helps in the identification and treatment of bacterial infections,2 while serial measurements of CRP are of prognostic value.3
The ongoing worldwide coronavirus disease-2019 (COVID-19) pandemic has posed an enormous threat to public health globally. It is caused by a type of coronavirus called severe acute respiratory syndrome coronavirus-2 (SARS-CoV-2)4 with which it shares an approximately 79% similarity at the nucleotide level. As of July 7, 2020, SARS-CoV-2 had caused 11,327,790 cases of COVID-19 infection with 532,340 deaths globally, with 719,665 cases and 20,160 deaths in India.5,6
The clinical severity of COVID-19 ranges from asymptomatic to pneumonia, acute respiratory distress syndrome (ARDS), and even death in humans.7 ARDS develops in severely affected patients due to diffuse alveolar damage. Therefore, continuous monitoring of the COVID-19 infection and effective early intervention are the basic measures for reducing the mortality rates due to this disease. Acute pulmonary inflammation could be a complex pathophysiological mechanism involving inflammatory mediators such as cytokines and chemokines, which stimulate the macrophages within the alveoli resulting in poor regulation of the system.8
In humans, the clinical progression of the novel coronavirus-induced disease occurs in 3 phases.9 The clinical features during the first phase include fever, dry cough, myalgia, and other systemic infections that are likely to be increased by the multiplication of the virus and the apoptosis induced by it. In the second phase, there is continuous replication of the virus leading to worsening of the symptoms and a drop in the immunoglobulin (IgG) levels. This could be due to severe damage to the alveoli caused by the host’s overt immune response.10-12 In novel coronavirus disease-19 (nCOVID19)-infected patients, one-third of the patients progress to the third phase, which is characterized by severe lung inflammation resulting in ARDS.12 Children, especially <12years old; the elderly population; and patients with comorbidities were reported to be excessively prone to the serious adverse outcomes of COVID-19 infection.12
Two Indian geneticists investigated 3600 strains of COVID-19 collected from 55 countries, which were observed to have a specific kind of virus referred to as Type 2a that has now replaced the original strain found in Wuhan. Their research showed that Type-O spread from China to other countries and later, it evolved within and outside China. The categories of 35 viral sequences are grouped into four types (Fig 1).13
Major Inflammatory Markers
Procalcitonin (PCT)
PCT is a protein made up of 116 amino acids and is the precursor of calcitonin. Calcitonin is a hormone synthesized by the parafollicular cells or C cells of the thyroid and is involved in calcium homeostasis. Endopeptidase-cleaved pre-procalcitonin produces PCT, which is a biomarker that can be detected within 3-4 h of an infection, following the release of tumor necrosis factor alpha (TNF-α) at 90 min and interleukin-6 (IL-6) at 3 h. It peaks at 6-12 h and has a half-life of about 24 h. This favorable kinetic profile and its specificity and sensitivity with regard to bacterial infections make it suitable for disease progression monitoring and identification of appropriate antibiotic treatment for individuals with bacterial sepsis, community-acquired pneumonia, suspected or confirmed lower respiratory tract infections, acute bronchitis, acute exacerbations of chronic obstructive pulmonary disease (COPD).14
Most COVID-19 patients have very low PCT levels (<0.1-0.25 µg/L) at the time of admission. Although elevated levels of inflammatory markers such as white blood count (WBC) and CRP indicate pulmonary inflammation, PCT could be a more potent tool for identifying COVID-19-positive individuals.15 It can be assessed quantitatively by paramagnetic particle chemiluminescent immunoassay.16
D-dimer
D-dimer, a product of fibrin degradation, is found in significantly elevated levels due to cytokine activation of the coagulation cascade in thrombotic patients.17 A shift in the alveolar hemostatic balance is observed in pulmonary infections.18
In patients with severe sepsis due to inflammatory conditions, cytokines cause endothelial injury, thereby activating coagulation and inhibiting fibrinolysis. Studies have shown that COVID-19 is associated with hemostatic abnormalities and elevated D-dimer levels were seen in non-survivors when compared to survivors.19 A retrospective cohort study conducted on 191 COVID19-positive patients found that D-dimer levels of >1.0 μg/ mL led to increased mortality (p=0.0033). Many studies have reported marked increase in the D-dimer levels in about 90% of the patients with pneumonia. Huang et al observed in their study that the quantity of D-dimer could be used for screening patients undergoing critical care. D-dimer was analyzed using monoclonal antibody-based and enzyme-linked immunosorbent assay (ELISA).21 The median D-dimer levels were increased in critically ill patients in ICU (2.4 mg/L vs 0.5 mg/L; p=0.0042) when compared to non-ICU patients.20 Thus, D-dimer is an important prognostic marker in monitoring COVID19-positive patients.
Lactate dehydrogenase (LDH)
LDH is a tetramer consisting of two subunits, LDH-M and LDH-H proteins, which are encoded by LDHA and LDHB genes, respectively. It is an intracellular enzyme found in almost all organ systems, involved in the conversion of pyruvate to lactate and vice versa and also linked to the interconversion of nicotinamide adenine dinucleotide hydrate (NADH) and NAD+ . LDH is present in humans in the form of five isoenzymes; LDH-1, which is present in cardiomyocytes; LDH-2 in the reticuloendothelial system, LDH-3 in pneumocytes, LDH-4 in kidneys and pancreas, and LDH-5 in liver and striated muscle.22 Since the 1960s, LDH has acted as a marker for cardiac damage. Abnormal values of LDH might be due to decreased oxygenation along with upregulation of the glycolytic pathway and multiple organ injury.
Whenever any infection or tissue injury occurs, the extracellular pH is altered due to a surge in the production of lactate that results in the activation of metalloprotease and enhancement of macrophage-mediated angiogenesis. Severe infections may cause cytokine-mediated tissue damage that leads to a surge in the LDH levels. As LDH3 occurs in the lungs, individuals with severe COVID-19 viral infection can be expected to have increased LDH levels in their circulation. Elevated LDH levels have also been detected in thrombotic microangiopathy, which is associated with myocardial injury, renal failure, hypothyroidism, and cancer. Several studies suggest that increased serum LDH can serve as a potential biomarker for evaluating serious idiopathic pulmonary fibrosis and monitoring the treatment response. Elevated LDH is considered a common sign of cellular or tissue damage. Patients with severe pulmonary interstitial disease show a significant increase in LDH levels and therefore, it is regarded as the most important prognostic markers of lung injury.23 Similarly, the amount of increase in LDH levels in critically ill patients infected with COVID-19 indicated the extent of lung injury. Serum LDH is estimated colorimetrically by measuring the concentration of Fe2+ complexed with 2,2-bipyridyl as Fe3+ is reduced to Fe2+ by the formation of NADH, when lactate is oxidized to pyruvate.24
C-reactive protein (CRP)
CRP is an acute-phase reactant which is produced in the liver and works as an important biomarker for the early detection of inflammation and infection.25 The normal concentration of CRP in serum is less than 10 mg/L; however, with the onset of disease, it elevates rapidly within 6-8 h and gives the highest peak in 48 h. The half-life of CRP is about 19 h and its concentration decreases with cessation of the inflammation or when the individual begins healing.26
When there is an inflammation or cellular damage, CRP binds to phosphocholine, which is expressed on the cell surface. This binding activates the classical complement pathway of the immune system that modulates the phagocytic activity to clear damaged cells and microbes. When the damage is resolved, the CRP concentration too decreases thus, making it a significant biomarker for monitoring the severity of the disease. Studies have shown that CRP is one of the significant markers in patients infected with COVID-19. On an average, the CRP level elevates to 20-50 mg/L in patients with COVID-19. A study has reported that in patients with mild-to-moderate symptoms, the CRP level had increased to 18.8 mg/L; however, patients with severe symptoms had an average concentration of 39.4 mg/L, and patients who died from COVID-19 had a 10-fold increase in the CRP concentration (46 mg/L). It has also been observed that patients with low oxygen saturation (SpO2 ≤90%) and severe lung damage have higher concentration of CRP.27
Increased CRP production is associated with overproduction of inflammatory cytokines, which fight against the microbes. However, when the immune system becomes hyperactive, it ends up damaging the lung tissue. Thus, CRP production is induced by inflammatory cytokines and tissue destruction in COVID-19 patients.28 Kinetic estimation of the concentration of CRP is performed by photometric measurement of antigen-antibody reaction between antibodies to human CRP bound to polystyrene particles and CRP present in the sample, at 546 nm or 700 nm.29
Ferritin
A molecule of ferritin consists of 24-peptide subunits. Ferritin is a protein that is expressed in numerous tissues and cell types and is also present in body fluids, particularly in blood plasma and serum. It is composed of two subunits—ferritin-heavy (feH) and ferritin-light (feL) subunits. These subunits are either catalytically active H subunits or catalytically inactive and specific to mammals - L subunits. Brain, kidney, and heart have H subunits, whereas liver and spleen express L subunits. Serum ferritin is mostly composed of L subunits, indicating its hepatic origin. The 24-peptide subunits form a hollow spherical shell that encases a core of iron. 30,31
Ferritin is also an example of an acute-phase protein whose concentration increases in infectious and non-infectious inflammations. As it can enhance the inflammatory process, an elevated level of ferritin is observed in COVID-19-infected individuals. A significant increase in serum ferritin concentration (2817.6 ng/mL) in severely infected patients has been demonstrated in a study.32 Macrophage activation syndrome (MAS) has been associated with high ferritin level. Severe COVID-19 infection is accompanied by cytokine storm syndrome. Thus, ferritin levels might be a crucial factor influencing the severity of COVID-19.33 It can be analyzed using immunoassays such as ELISA, chemiluminescence, and immunoturbidimetry.34
Cytokine profile
The human body’s defense mechanism is capable of responding to various pathogens. A viral infection leads to metabolic dysfunctions, which requires reprogramming of the host metabolism to trigger antiviral defense responses. Cytokines are polypeptide molecules mainly used to regulate biological process through cell surface receptors.35 Cytokines are produced by immune cells and are a necessary part of the inflammatory process. The pro-inflammatory cytokines include IL1, IL-6, IL-17, TNF-α, and interferon (IFN). Antiinflammatory cytokines comprise IL-10 and cytokines which are involved in adaptive immunity–IL-2 and IL-4. Cytokines are secreted as a defensive response to stress (eg, microbial infection).36
Several studies have reported that during COVID-19 infection, there is release of a large amount of proinflammatory cytokines, producing an aggressive inflammatory response resulting in a “cytokine storm.”37 It is caused by a sudden acute increase in the circulating levels of various pro-inflammatory cytokines of the innate immune response, including IL-1, IL-6, TNF-α, and interferon. This increase causes an influx of various immune cells such as macrophages, neutrophils, and T-cells from the circulation to the site of infection, with destructive effects such as capillary damage, vascular barrier damage, diffuse alveolar damage, multi-organ failure, and mortality. After analyzing the cytokine profiles of COVID-19 patients, it has been suggested that the cytokine storm is directly correlated with lung injury and multi-organ failure. Other cytokines and chemokines, which have also been reported to increase with the onset of COVID-19 infection, are IL-7, IL-12, IL-13, macrophage colony-stimulating factor (M-CSF), granulocyte colony-stimulating factor (G-CSF), granulocyte-macrophage colony-stimulating factor (GM-CSF), interferon gamma-induced protein (IP-10), hepatocyte growth factor (HGF), and vascular endothelial growth factor (VEGF). SARS-CoV-2 infection impedes antiviral defenses and produces more inflammatory cytokines.
Both ELISA and enzyme-linked immunospot (ELISPOT) assays are used to detect cytokines in single cells and are indirect methods for identifying gene expression for cytokine production by polymerase chain reaction (PCR). The fluorospot assay is a modification of the ELISPOT assay which depends on enzyme action instead of the bound fluorophore. These assays are now used for estimating two different cytokines in the same cell by using different anti-cytokine antibodies.38
Pro-inflammatory Cytokines
Interleukin-1 (IL-1)
IL-1 plays a vital role in the pathogenesis of acute and chronic obstructive respiratory diseases. It is produced by immune cells, endothelial cells, and fibroblasts at the site of inflammation in response to the pathogenic virus. When IL-1 is stimulated, it causes fever, inflammation of tissues, and fibrosis.
Macrophages activated by COVID-19 perform phagocytic activity on the debris of dead cells and tissues, releasing inflammatory substances and thus, are crucial for the pathogenesis of fibrosis.39 These reactions are related to danger-associated molecular patterns, which in turn have pattern recognition receptors including toll-like receptors (TLRs). Activation of several TLRs, such as TLR2, TLR3, or TLR4, leads to the release of inflammatory cytokines such as IL-1β. In COVID-19 infection, IFN type I is essentially produced, which has various effects on both innate and acquired immunities but above all, against viral infections.`40 However, there are certain harmful effects, such as inflammation, apoptosis, tissue damage, in some cases suppression of the immune system, and cell proliferation, due to the IFN production.
Interleukin-6 (IL-6)
The IL-6 gene is located on chromosomes 7p21 and 5 in the human and mouse genomes, respectively. The human, mouse, and rat IL-6 gene has been cloned and sequenced. It contains four introns and five exons. Within the protein-coding region of the gene, the positions of the exon/intron boundaries, exon lengths, and location of cysteine residues within exons are conserved across species.
Recent studies have shed light on the importance of serum IL-6 as a diagnostic biomarker in COVID-19 patients. It is a pro-inflammatory mediator, which induces acute-phase response and autoimmune injury. It has been observed that excessive synthesis of IL-6 causes exaggerated inflammatory responses which lead to severe acute systemic inflammatory response cytokine storm. Inflammatory cytokine storm is one of the main causes of COVID-19 pathogenesis, as well as the resulting mortality.41
The SARS-CoV-2 enters cells by interacting with angiotensin-converting enzyme (ACE) in the target tissues such as lung, heart, kidney, and gastrointestinal tract (Fig 3). It attacks the alveolar epithelial cells of the lungs, leading to severe destruction and permeability of epithelial cells following which, the virus is released. The inflammatory sensors sense the viral RNA and its structural proteins, and activate the inflammatory cascade including inflammatory cytokines such as IL-6 and inflammasomes.42 Additionally, a large number of inflammatory cells and erythrocytes enter the alveoli, causing dyspnea and respiratory failure. Correspondingly, there is an increase in the serum IL-6 level, suggesting that it is remarkably associated with the severity of patients’ clinical manifestations. It was observed that IL-6 was significantly increased in severely infected patients, but not in mild cases.43
Tumor necrosis factor alpha (TNF-α)
TNF-α is initially synthesized as a 26 kDa precursor molecule that is subsequently processed to the mature form by cleavage of the Ala-76 – Val-77 bond. The 17 kDa carboxyl-terminal protein is then secreted to function in a paracrine manner. The enzyme that processes the TNF-α precursor has previously been identified as a microsomal metalloprotease called TNF-α converting enzyme (TACE).44 TNF-α is produced by various cell types such as monocytes, macrophages, and T-cells. This cytokine has been associated with proinflammatory responses mediated by IL-1β and IL-6 and has been reported to be increased in the blood of COVID-19 patients. Diao et al reported similar results in a sample of 522 COVID-19 patients and observed an inverse relationship between the TNF-α levels and T-cell counts. In contrast, Wan et al described normal TNF-α levels in patients with COVID-19.45 It is one of the cytokines whose overproduction is associated with poor prognosis in patients with SARS-CoV-2 and Middle East respiratory syndrome (MERS).
Interferon gamma (IFN-γ)
The IFNγ monomer is made up of a core of six α-helices and in the C-terminal region extended unfolded sequences. IFN-γ is a type-2 IFN produced in numerous cells. It is mainly produced by T- and NK cells, as well as by lymphocyte cells including CD4+ and CD8+ T-cells, Treg cells, FoxP3+, CD8-, T-cells, and B-cells.46 This cytokine is also produced by macrophages, dendritic cells, monocytes, and neutrophil granulocytes. It takes part in inflammatory processes and is involved in signal transduction, and antibacterial and antiviral immunity. Previous studies have reported that the IFN-γ levels were higher in SARS-CoV-2 and MERS patients.
Huang et al noted that serum IFN-γ levels were elevated in patients with COVID-19 when compared to healthy individuals, which was due to the activation of Th1 and Th2 cells. Liu et al (2020) reported that elevated IFN-γ levels were associated with greater viral load and lung damage. Sun et al found that IFN-γ, IL-6, and IL-10 concentrations were increased in individuals infected with SARS-CoV-2 but were unchanged in both ICU and non-ICU patients.47 These authors established that IFN-γ levels were lower in CD4+ T-cells in infected patients with severe against mild symptoms and also demonstrated that the CD4+ and CD8+ T-cells, reducing the assembly of IFN-γ affected at the starting stage of the infection.
Anti-inflammatory Cytokines
Interleukin-10 (IL-10)
IL-10 is an intercalated dimer of two subunits consisting of six amphipathic helices A-F. The polypeptide chains of each subunit contribute to both parts of the dimer. Helices A-D of one subunit form a distinctive six-helix domain with helices E and F through 180° rotation around two-fold axis.48 IL-10 is found to be elevated in COVID-19 patients compared to SARS-CoV-2 or MERS. There is an increased expression of IL-10 along with increase in disease severity. It is produced by a variety of cells, including endothelial cells, epithelial cells, macrophages, lymphocytes, and fibroblasts at the site of tissue inflammation during acute organ injuries and sepsis.49 IL-10 is an anti-inflammatory cytokine, which inhibits the production of pro-inflammatory cytokines. It blocks IL-12 and inhibits the maturation of dendritic cells. It plays a vital role in regulating host immune response to infections, and in the proliferation and differentiation of B-cells, thymocytes, and mast cells. IL-10 regulation is hampered during a viral infection, resulting in depletion of T-cells. Numerous studies have demonstrated that IL-10 is overexpressed in anti-SARSCoV-2 immunity. As it was observed to be elevated only in severe cases of viral infection, the inhibitory role of this cytokine is likely related to the overall suppression of the defense mechanism, viral control, and disease severity.50 One of the studies reported that although the concentration of IL-10 was high (25.66 pg/mL), the symptoms of the infected patient were moderate.
Cytokines in Adaptive Immunity
Interleukin-2 (IL-2)
IL-2 is an interleukin, one of the signaling molecules, which is involved in the defense of the body. It causes a build-up of about 15.5-16 kDa protein that controls the activities of white blood corpuscles (leukocytes, often lymphocytes) which are very crucial for immunity. It plays a major role in fighting against microbial infections and also helps in differentiating between native and foreign particles. Lymphocytes produce IL-2 receptors which will be bounded by IL-2. IL-2 is mainly produced by activated T-cells; therefore, elevated IL-2 concentration reflects T-cell activation.51 It plays a key role in adaptive immunity; helps in proliferation and activation of T-, B-, and NK cells; and increases glucose metabolism. Absence of IL-2 is almost similar to autoimmunity and poor control of effector T-cells. Huang et al reported that IL-2 or its receptor IL-2R is elevated in COVID-19-infected patients. The increase in the levels of this interleukin is directly proportional to the severity of the disease.52 The concentration of IL-2 reached its peak in 15-20 days followed by moderate decline in severe cases of infection, whereas no significant difference was noted in mild cases.
Interleukin 4 (IL-4)
IL-4 is a cytokine that causes the differentiation of helper T-cells (Th0 cells) to Th2 cells. When Th2 cells are activated by IL-4, they produce more IL-4 in a positive feedback loop. The cells that produce IL-4 have not been identified, but most of the recent studies suggest that basophils might be acting as effector cells. IL-4 is very similar to IL-13 and also has similar functions. It is involved in adaptive immunity and plays a crucial role in the activation, proliferation, and differentiation of B-lymphocytes. It also helps in inducing humoral immunity and enhancing immunoglobulin E production. The anti-inflammatory function of IL-4 is extremely tissue-specific, indicating the metabolic plasticity of different tissues. It was observed that IL-4 was remarkably increased in mild patients but not in severely infected patients. Bot et al suggested that IL-4 has negative effects on CD8+ memory T-cells during viral infections. Various studies have concluded that IL-4 has a built-in cytokine storm and is elevated in COVID-19 patients, causing severe respiratory symptoms.
Conclusion
In majority of the cases, the immunopathogenesis of nCOVID-19 infection involves disturbances in the inflamatory mediators that do not actually cause the disease but play a pivotal role in its progression. This review has highlighted the various strains of coronaviruses and also described the pathogenesis of various inflamatory markers or profiles, which would be helpful in developing an informative guide for planning therapeutic interventions.
Conflict of Interest
None.
Supporting File
References
- Schuetz P, Beishuizen A, Broyles M, Ferrer R, Gavazzi G, Gluck EH, et al. Procalcitonin (PCT)- guided antibiotic stewardship: an international experts consensus on optimized clinical use. Clin Chem Lab Med. 2019;57(9):1308-18.
- Meisner M. Update on procalcitonin measurements. Ann Lab Med. 2014;34(4):263-73.
- Lelubre C, Anselin S, Boudjeltia KZ, Biston P, Piagnerelli M. Interpretation of C-reactive protein concentrations in critically ill patients. Biomed Res Int. 2013;2013:124021.
- Liu F, Li L, Xu M, Wu J, Luo D, Zhu Y, et al. Prognostic value of interleukin-6, C-reactive protein, and procalcitonin in patients with COVID-19. J Clin Virol. 2020;127:104370.
- World Health Organization. Coronavirus disease (COVID-19) Situation Report – 168. 2020. Available from: https://www.who.int/docs/defaultsource/coronaviruse/situation-reports/20200706- covid-19-sitrep-168.pdf?sfvrsn=7fed5c0b_2
- Government of India. Ministry of Health and Family Welfare. Novel Coronavirus Updates. 2020. Available from: https://www.mohfw.gov.in
- Chen L, Liu HG, Liu W, Liu J, Liu K, Shang J, et al. Analysis of clinical features of 29 patients with 2019 novel coronavirus pneumonia. Zhonghua Jie He He Hu Xi Za Zhi. 2020;43(0):E005.
- Guan WJ, Ni ZY, Hu Y, Liang WH, Ou CQ, He JX, et al. Clinical Characteristics of Coronavirus Disease 2019 in China. N Engl J Med. 2020;382(18): 1708-20.
- Nicholls JM, Poon LLM, Lee KC, Ng WF, Lai ST, Leung CY, et al. Lung pathology of fatal severe acute respiratory syndrome. Lancet. 2003;361(9371):1773-8.
- Huang KJ, Su IJ, Theron M, Wu YC, Lai SK, Liu CC, et al. An interferon-gamma-related cytokine storm in SARS patients. J Med Virol. 2005;75(2):185-94.
- Heugel J, Martin ET, Kuypers J, Englund JA. Coronavirus-associated pneumonia in previously healthy children. Pediatr Infect Dis J. 2007;26(8):753-5.
- Covid-19 evolution: one strain overwhelms others Available from: https://www.deccanherald. com/national/covid-19-evolution-one-strainoverwhelms-others-831139.htm
- Kogan AE, Mukharyamova KS, Bereznikova AV, Filatov VL, Koshkina EV, Bloshchitsyna MN, et al. Monoclonal antibodies with equal specificity to D-dimer and high-molecular-weight fibrin degradation products. Blood Coagul Fibrinolysis. 2016;27(5):542-50.
- Favaloro EJ, Jennings I, Olson J, Van Cott EM, Bonar R, Gosselin R, et al. Towards harmonization of external quality assessment/proficiency testing in hemostasis. Clin Chem Lab Med. 2018;57(1): 115-26.
- Crawford F, Andras A, Welch K, Sheares K, Keeling D, Chappell FM. D-dimer test for excluding the diagnosis of pulmonary embolism. Cochrane Database Syst Rev 2016;2016(8):CD010864.
- Wacker C, Prkno A, Brunkhorst FM, Schlattmann P. Procalcitonin as a diagnostic marker for sepsis: a systematic review and meta-analysis. Lancet Infect Dis. 2013;13(5):426-35.
- Linkins L-A, Lapner ST. Review of D-dimer testing: Good, Bad, and Ugly. Int J Lab Hematol. 2017;39(1):98-103.
- Yao Y, Cao J, Wang Q, Shi Q, Liu K, Luo Z, et al. D-dimer as a biomarker for disease severity and mortality in COVID-19 patients: a case control study. J Intensive Care. 2020;8:49.
- Sakka M, Connors JM, Hékimian G, MartinToutain I, Crichi B, Colmegna D, et al. Association between D-Dimer levels and mortality in patients with coronavirus disease 2019 (COVID-19): a systematic review and pooled analysis. J Med Vasc. 2020;45(5):268-74.
- Henry BM, Aggarwal G, Wong J, Benoit S, Vikse J, Plebani M, et al. Lactate dehydrogenase levels predict coronavirus disease 2019 (COVID-19) severity and mortality: A pooled analysis. Am J Emerg Med. 2020;38(9):1722-6.
- Riley RS, Gilbert AR, Dalton JB, Pai S, McPherson RA. Widely Used Types and Clinical Applications of D-Dimer Assay. Lab Med. 2016;47(2):90-102.
- Khan AA, Allemailem KS, Alhumaydhi FA, Gowder SJT, Rahmani AH. The Biochemical and Clinical Perspectives of Lactate Dehydrogenase: An Enzyme of Active Metabolism. Endocr Metab Immune Disord Drug Targets. 2020;20(6):855-68.
- Yan L, Zhang H, Mendes Silva Goncalves J, Xiao Y, Wang M, Guo Y, et al. An interpretable mortality prediction model for COVID-19 patients. Nat Mach Intell. 2020;2(5):283-8.
- Kjeld M. An automated colorimetric method for the estimation of lactate dehydrogenase activity in serum. Scand J Clin Lab Invest. 1972;29(4):421-5.
- Wang L. C-reactive protein levels in the early stage of COVID-19. Med Mal Infect. 2020;50(4):332-4.
- Ali N. Elevated level of C-reactive protein may be an early marker to predict risk for severity of COVID-19. J Med Virol. 2020;92(11):2409-11.
- Herold T, Jurinovic V, Arnreich C, Lipworth BJ, Hellmuth JC, von Bergwelt-Baildon M, et al. Elevated levels of IL-6 and CRP predict the need for mechanical ventilation in COVID-19. J Allergy Clin Immunol. 2020;146(1):128-36.
- Kappert K, Jahić A, Tauber R. Assessment of serum ferritin as a biomarker in COVID-19: bystander or participant? Insights by comparison with other infectious and non-infectious diseases. Biomarkers. 2020;25(8):616-25.
- Osmond AP, Friedenson B, Gewurz H, Painter RH, Hofmann T, Shelton E. Characterization of C-reactive protein and the complement subcomponent C1t as homologous proteins displaying cyclic pentameric symmetry (pentraxins). Proc Natl Acad Sci USA. 1977;74(2):739-43.
- Ruscitti P, Berardicurti O, Di Benedetto P, Cipriani P, Iagnocco A, Shoenfeld Y, et al. Severe COVID-19, Another Piece in the Puzzle of the Hyperferritinemic Syndrome. An Immunomodulatory Perspective to Alleviate the Storm. Front Immunol. 2020;28(11):1130.
- Vargas-Vargas M, Cortés-Rojo C. Ferritin levels and COVID-19. Rev Panam Salud Publica. 2020;44:e72.
- Bartee E, McFadden G. Cytokine synergy: an underappreciated contributor to innate anti-viral immunity. Cytokine. 2013;63(3):237-40.
- Turner MD, Nedjai B, Hurst T, Pennington DJ. Cytokines and chemokines: At the crossroads of cell signalling and inflammatory disease. Biochim Biophys Acta. 2014;1843(11):2563-82.
- Garcia-Casal MN, Peña-Rosas JP, Urrechaga E, Escanero JF, Huo J, Martinez RX, et al. Performance and comparability of laboratory methods for measuring ferritin concentrations in human serum or plasma: A systematic review and meta-analysis. PLoS One. 2018;13(5):e0196576.
- Vabret N, Britton GJ, Gruber C, Hegde S, Kim J, Kuksin M, et al. Immunology of COVID-19: Current State of the Science. Immunity. 2020;52(6):910-41.
- Ye Q, Wang B, Mao J. The pathogenesis and treatment of the ‘Cytokine Storm’ in COVID-19. J Infect. 2020;80(6):607-13.
- Conti P, Ronconi G, Caraffa A, Gallenga C, Ross R, Frydas I, et al. Induction of pro-inflammatory cytokines (IL-1 and IL-6) and lung in flammation by Coronavirus-19 (COVI-19 or SARS-CoV-2): anti-inflammatory strategies. J Biol Regul Homeost Agents. 2020;34(2):327-31.
- Stenken JA, Poschenrieder AJ. Bioanalytical chemistry of cytokines--a review. Anal Chim Acta. 2015;853:95-115.
- Chen C, Zhang XR, Ju ZY, He WF. Advances in the research of mechanism and related immunotherapy on the cytokine storm induced by coronavirus disease 2019. Zhonghua Shao Shang Za Zhi. 2020;36(6):471-5.
- Liu Y, Yang Y, Zhang C, Huang F, Wang F, Yuan J, et al. Clinical and biochemical indexes from 2019- nCoV infected patients linked to viral loads and lung injury. Sci China Life Sci. 2020;63(3):364-74.
- Zhou Y, Fu B, Zheng X, Wang D, Zhao C, qi Y, et al. Pathogenic T cells and inflammatory monocytes incite inflammatory storms in severe COVID-19 patients. Natl Sci Rev. 2020;7(6):998-1002.
- Heinrich PC, Castell JV, Andus T. Interleukin-6 and the acute phase response. Biochem J. 1990;265(3):621-36.
- Rose-John S. IL-6 trans-signaling via the soluble IL-6 receptor: importance for the pro-inflammatory activities of IL-6. Int J Biol Sci. 2012;8(9):1237-47.
- Moss ML, Jin SL, Becherer JD, Bickett DM, Burkhart W, Chen WJ, et al. Structural features and biochemical properties of TNF-alpha converting enzyme (TACE). J Neuroimmunol. 1997;72(2): 127-9.
- Thiel DJ, le Du MH, Walter RL, D›Arcy A, Chène C, Fountoulakis M, et al. Observation of an unexpected third receptor molecule in the crystal structure of human interferon-gamma receptor complex. Structure. 2000;8(9):927-36.
- Diao B, Wang C, Tan Y, Chen X, Liu Y, Ning L, et al. Reduction and Functional Exhaustion of T Cells in Patients with Coronavirus Disease 2019 (COVID-19). Front Immunol. 2020;11:827.
- Dhama K, Patel SK, Pathak M, Yatoo MI, Tiwari R, Malik YS, et al. An update on SARS-CoV-2/ COVID-19 with particular reference to its clinical pathology, pathogenesis, immunopathology and mitigation strategies. Travel Med Infect Dis. 2020;37:101755.
- Zdanov A, Schalk-Hihi C, Wlodawer A. Crystal structure of human interleukin-10 at 1.6 A resolution and a model of a complex with its soluble receptor. Protein Sci. 1996;5(10):1955-62.
- Sampaio EP, Sarno EN, Galilly R, Cohn ZA, Kaplan G. Thalidomide selectively inhibits tumor necrosis factor alpha production by stimulated human monocytes. J Exp Med. 1991;173(3):699-703.
- Arenas-Ramirez N, Woytschak J, Boyman O. Interleukin-2: Biology, Design and Application. Trends Immunol. 2015;36(12):763-77.
- Liao W, Lin J-X, Leonard WJ. IL-2 family cytokines: new insights into the complex roles of IL-2 as a broad regulator of T helper Wcell differentiation. Curr Opin Immunol. 2011;23(5):598-604.
- Silva-Filho JL, Caruso-Neves C, Pinheiro AAS. IL4: an important cytokine in determining the fate of T cells. Biophys Rev. 2014;6(1):111-8.