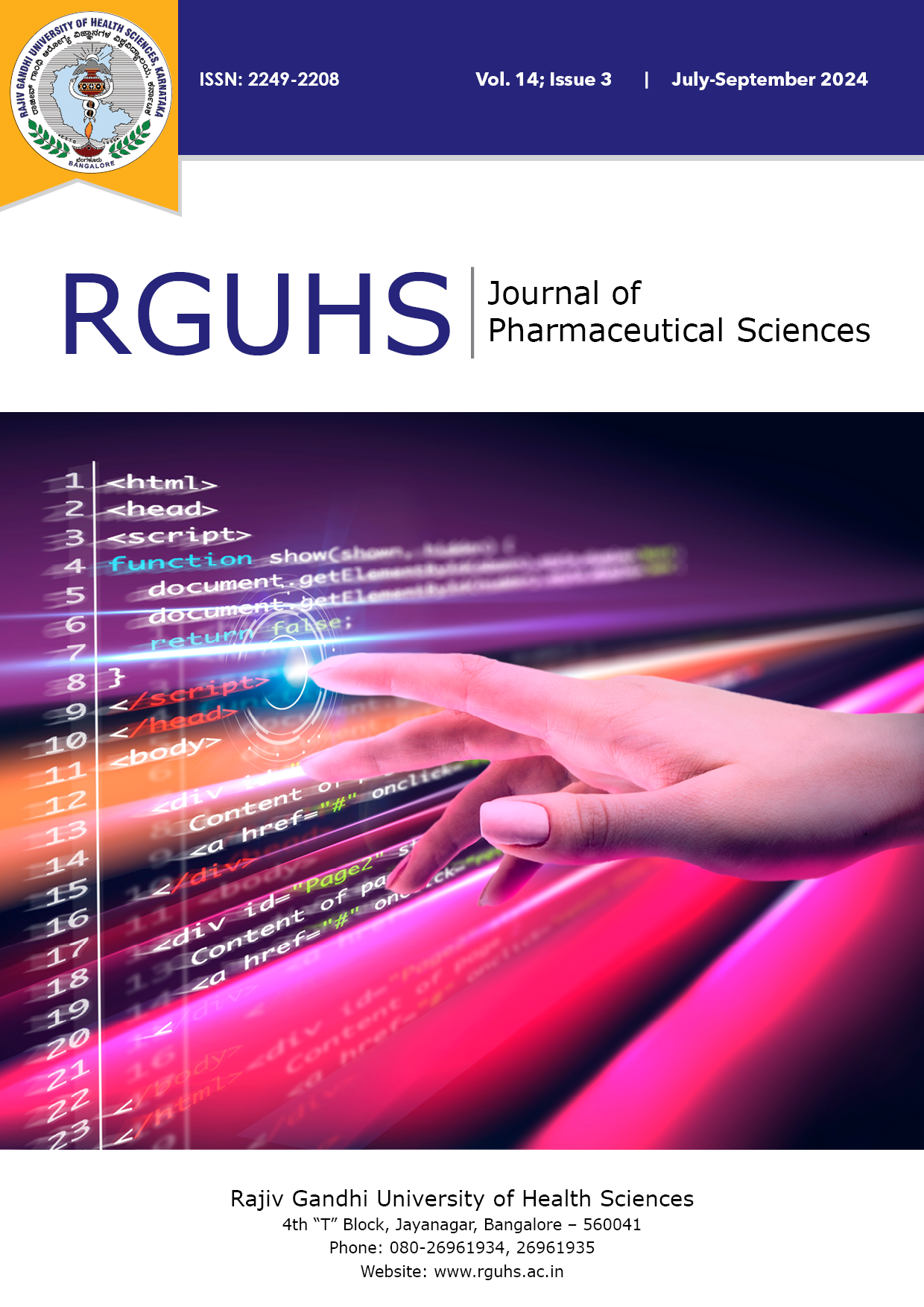
RJPS Vol No: 14 Issue No: 3 eISSN: pISSN:2249-2208
Dear Authors,
We invite you to watch this comprehensive video guide on the process of submitting your article online. This video will provide you with step-by-step instructions to ensure a smooth and successful submission.
Thank you for your attention and cooperation.
Ashwini M1*, Preethi Sudheer2 , Bharani S Sogali3
1,2Department of Pharmaceutics, Krupanidhi College of Pharmacy, Chikkabellandur Post, Bangalore, Karnataka - 560035. 3 Healthcare Hospital, Kolar, Karnataka – 5631101.
*Corresponding author:
Ashwini M, Research Scholar, Krupanidhi College of Pharmacy, Chikkabellandur Post, Bangalore, Karnataka - 560035. E-mail: ashwinipreetham2@gmail.com
Received date: February 16, 2022; Accepted date: March 13, 2022; Published date: March 31, 2022

Abstract
Background: Nanostructured lipid carriers (NLCs) are lipid form of nanoparticles with an unstructured solid and liquid lipid core that allows for the intercalation of hydrophilic/lipophilic drugs, guarding them from deterioration and increasing their stability. NLC is a current evolution of colloidal vehicles composed of lipids, water, and surfactants that allow for the elimination of many of the constraints and disadvantages of older lipoidal systems.
Aim of the study: The most important characteristics that distinguish NLC from first-generation solid lipid nanoparticles are greater drug loading and higher stability (SLN). In the current study, glibenclamide NLCs were synthesised using the hot homogenization technique with ultrasonication and then subjected to stability studies. The lipid ratio optimization was done using a custom design with the help of JMP pro-13.
Methodology: Stability studies of prepared NLCs were assessed by keeping the formulation in sealed glass containers at 25±2ºC / 60±5% RH and 40±2ºC / 75±5% RH for six months, and then evaluated for various parameters to inscribe changes.
Results: The results showed no significant changes in the NLCs during the six months of its storage period at the specified conditions.
Conclusion: Hence, it may be inferred that prepared NLCs were stable and room temperature could be the suitable condition for storage of the prepared glibenclamide NLCs
Keywords
Downloads
-
1FullTextPDF
Article
Introduction
Nanotechnology is widely used in the administration of drugs in a variety of dosage forms. Nanoparticles are colloidal particulate formations whose size ranges from 10 to 1000 nanometers in diameter. In the early nineties, solid lipid nanoparticles (SLNs) were presented as a novel formulation with a number of distinct advantages, including the utilization of biocompatible lipids, the use of a few organic solvents during formulation, good in vivo stability, and a broad utilization spectrum. SLNs are composed of solid lipids, surfactants, and active ingredients. Nonetheless, low drug loading potential, unpredictability of gelation, polymorphic transformation, and drug leakage upon storage are some of the constraints associated with SLNs. To address the shortcomings of first-generation solid lipid nanoparticles, nanostructured lipid carriers (NLCs) were developed as the next inception lipid nanoparticles. Bio– based, degradable and amicable lipids (solid and liquid) are used to formulate NLCs. Further, the addition of liquid lipids (oil) results in structural flaws in solid lipids, culminating in a less ordered structure that curtails drug seepage while providing an appreciable drug load.1
The present study focused on preparation of NLCs of glibenclamide (GB), exploring the stability aspect of the same. Glibenclamide is a sulfonylurea, hypoglycemic agent of the second generation taken orally. It is useful when there is partial release of insulin from the pancreas. In a recent study, the rate of maternal hypoglycemia in women who received insulin was higher than in women who received glibenclamide.2 When compared to insulin, glibenclamide has a comparable rate of success in achieving target glucose levels, improved pregnancy outcomes, and significantly fewer hypoglycemic episodes. Also, glibenclamide has lower maternal-tofetal transit than first-generation hypoglycemic drugs. Loading glibenclamide to NLCs increases its stability and gives the flexibility to explore alternate delivery option of administering GB, like transdermal route. In the present study, the stability feature of NLC loaded with GB was investigated with respect to size and distribution, electrostatic stability, particle morphology, drug content, and in vitro drug release.3
Materials and Methods
Mankind Pharma, India provided a free sample of glibenclamide. The oleic acid and stearic acid were provided as gift samples by Venus Ethoxyethers, Goa. All the other compounds and reagents were analytically pure.
Formulation of NLCs
The NLCs were prepared using a combination of homogenization and ultrasonication. The technique was performed at a temperature above the melting point of the lipids. Glibenclamide was mixed into molten stearic acid. The drug dispersion in molten solid lipid was diffused slowly into hot oleic acid (liquid lipid) and then streamed to aqueous phase that included the surfactant Tween 20, which was kept at about the same temperature (above 69.3°C) with continuous agitation. The resulting dispersion was then ultrasonicated and homogenised with a polytron homogenizer. Furthermore, NLCs were obtained by pouring this fine emulsion into a petri dish and allowing it to cool.4
Infrared spectroscopy
Infrared spectra of pure glibenclamide and prepared nanostructured formulations were determined using Bruker Alpha IR spectrometer to ascertain the compatibility between the drug and the excipients. The pure drug, and the formulations were scanned eight times on an attenuated total reflection (ATR) platform.5
Employment of central composite design for optimization of lipid ratio In NLC, solid and liquid lipids assemble the inner nucleus and according to the literature, the solid lipid and liquid lipid are mixed in a wide ratio. Lipid concentrations play a critical role in the success of the NLC formulation, as it affects the particle size, drug entrapment, drug release, and stability. Hence, central composite design (CCD) was used to optimise the solid and liquid lipid concentrations in drug-loaded NLCs. The central composite design possesses certain specified arrangement with a centre point surrounded by corner points and extra points bounded from the sides. The ratio parameters were optimized with two center points using software JMP pro 13. The design generated 10 experimental trials as indicated in the Table 1. The attributes particle size and drug encapsulation efficiency were investigated so as to determine the optimal solid-liquid lipid ratio.6 Based on the optimal ratio generated by the design, the GB-NLC was formulated and subjected to stability studies.
Stability study
The study was assessed in compliance with ICH recommendations. NLCs containing glibenclamide were evaluated for their stability by storing the samples at 25±2ºC / 60 ± 5% RH and 40±2 ºC / 75± 5% RH for 180 days in a stability chamber (Thermo Lab, Mumbai, India). The temperature scale of stability chamber ranged from 0 to 60°C that can be set in 0.1°C increments, the relative humidity, ranged from above ambient to 95 ±5.0% RH. The particles were evaluated for parameters like size analysis, zeta potential, morphology, drug entrapment and in-vitro drug release at the first day of storage and at the end of three months and six months. 7
Evaluation of nanostructured lipid carriers
Particle Size and zeta potential
To evaluate particle size and zeta potential, the HORIBA SZ-100 scientific analyser was deployed. By using the principle of dynamic light scattering technique, the size of the nanoparticles and particle size distribution were estimated. The electrostatic light scattering concept was used to compute the zeta potential. The samples were analysed after diluting with double distilled water at an angle of 90 degrees at a temperature of 25.2ºC.8
Scanning electron microscopy
This technique was used to evaluate the topography of the preparations (SEM). Gold-sputtered NLC dispersion was dispersed over a specimen chamber. 30 kV voltage and argon purging were used to capture the images.9
Atomic force microscopy (AFM)
AFM generates a three-dimensional topography with a fixed scan and provides a greater understanding of a surface. AFM scans the surface of the sample with a tip that moves up and down depending on the nature of the surface. It consists of a pointed tip with a diameter of 10 to 20 nm appended to a cantilever. AFM tips and cantilevers are made of silicon or silicon nitride. The tip moves as a result of tip-surface interactions, which is measured by fixating a laser beam with a photodiode.10
Drug encapsulation efficacy To compute the encapsulation effectiveness, the amount of glibenclamide present in the resultant supernatant was determined. NLC suspension containing 5 mg drug was centrifuged at a speed of 4000 rpm for a period of 45 minutes, to isolate the encapsulated and unencapsulated glibenclamide. The supernatant was diluted with methanol before being filtered, and the amount of unentrapped drug was measured spectrophotometrically at a wavelength of 300 nm. The percentage entrapment efficacy (EE%) was calculated using the below mentioned equation.11
Wa represents the total drug content of the NLC used in the study (equal to 5 mg), and Wb represents the extent of free drug in the supernatant after centrifugation.
In-vitro release profile
To study the in-vitro release, Franz diffusion cell was used. A semipermeable cellophane membrane of 3.14 cm2 area was placed between the donor and receptor chambers. Phosphate buffer solution pH 7.4 (PBS) was placed in the receptor section and kept at 37 ± 0.5°C and 600 rpm. In the donor chamber, NLC containing 5 mg glibenclamide was dispersed in phosphate buffer pH 5.5. Samples (1 ml) were discharged at frequent intervals and replenished with fresh buffer to maintain the sink scenario. UV spectroscopy at a maximum wavelength of 300 nm was used to ascertain the glibenclamide content of the samples. The release studies were carried out in triplicate.12
Results
In this investigation, NLCs were formulated with the lipids, stearic acid (solid lipid) and oleic acid (lipid liquid) by hot homogenization method. The nanoparticles were evaluated for multiple characteristics such as size, electrostatic zeta potential, drug encapsulation, morphology, and in-vitro release. Furthermore, the formulated nanoparticles were assessed for stability in a stability chamber by storing and sealing them in tightly closed containers at 25±2ºC / 60 ± 5% RH and 40±2ºC / 75± 5% RH for 180 days.
Infrared spectroscopy
IR studies were carried out for pure glibenclamide and NLC formulation as depicted in Figure 1. According to the findings of the infrared spectra of a number of sulphonyl urea derivatives, assignments for the peaks observed for glibenclamide can be made as follows: 3562.25 cm-1 to 3411.34 cm-1 due to N-H stretching, 1620.80 cm-1 to 1550 cm-1 due to amide C=O stretch, 1412.28 cm-1 due to N-H bending, 1318.57 cm-1 due to S=O stretching, 1124.22 cm-1 due to C-O stretch, 931.78 cm-1 due to C- Cl stretch.
Ratio optimization
The particle size varied in ten formulations from 60±0.546 to 637±0.087 nm illustrating the influence of changing the solid-liquid lipid ratio in glibenclamide NLC. Drug entrapment was determined indirectly and it varied from 60±1.00 to 90±0.58 percent. The results of size, and drug encapsulation are briefed in Table 1. Figure 2 gives the actual vs predicted plots and the p values obtained were less than 0.05 for both particle size and drug entrapment. The prediction profiler as indicated in Figure 3 gave the optimum proportion of solid and liquid lipid to be incorporated in the NLCs.
Particle size analysis and zeta potential
The size analysis data of optimised NLC was depicted in Figure 4. For samples stored at room temperature, there was no considerable variation at the end of 3 and 6 months. At the end of 6 months, the particles held at 40±2ºC / 75±5% RH showed a modest increase in particle size and the PDI was rather broader, as revealed in Figure 5. There was also an extra peak at the end of the six-month period. The zeta potential of NLC stored at room temperature did not show any variation, whereas NLCs stored at 40±2ºC / 75±5% RH showed a deviation of -25±1.05 at the end of six months as indicated in Table 3.
Scanning electron microscopy
The NLCs stored at stability study conditions were subjected to SEM to depict the changes in surface morphology and are illustrated in figure 6.
Atomic force microscopy
Atomic force microscopy is prevalently used to gather information about the size, shape, and surface morphological characteristics of nanoparticles. AFM images of NLCs stored for 6 months at 25 ±2 ºC / 60 ± 5% RH and 40±2 ºC / 75± 5% RH are show in Figure 7.
Drug content
The nanoparticles were observed for drug leakage, NLCs were placed at different storage conditions and periods. The quantity of residual drug in NLC at room temperature and at accelerated conditions were found to be 94.9±1.55% and 92.8±1.22% respectively at the end of six months and depicted in the Table 3.
In-vitro drug release studies
There was no notable change in the in-vitro release from NLCs stored at 25±2°C/60±5% RH for a period of six months as indicated in the Table 3. However, after storing the NLCs at 40±2 ºC / 75 ± 5% RH, the in vitro release decreased from 95.23±1.5% to 87.33±2.5% after 6 months.
Discussion Infrared spectroscopy
FTIR spectrum of glibenclamide was in correlation with the reference spectrum with peaks of all functional groups. The FTIR spectrum of the formulated NLC had the characteristic peaks and band values of glibenclamide and pure lipids, hence confirming that all the functional groups were well preserved, thus illustrating that there was no interaction between the drug and the chosen lipids.
Ratio optimization
From the mathematical prediction expression shown below for particle size, a positive value suggests that there will be increase in the response as the factor levels are increased, whereas a negative value specifies a converse relationship between the factor and the response. It is clear from the equation (1) that solid as well as liquid lipid concentration showed a negative effect on the particle size. Increase in the lipid concentration decreases the particle size.
78.71+-55.8333.solid lipid +-114.83333.liquid lipid+ solid lipid.(liquid lipid.165.25)+solid lipid.( solid lipid. 107.0714) +liquid lipid. (liquid lipid.115. 07142857) --- -eq 1
For the drug entrapment, liquid lipid (eq 2) concentration showed a negative impact whereas solid lipid concentration had a positive effect. 78.642857143+1.5.solid lipid-3.833333.liquid lipid+ solid lipid.(liquiid lipid.1.5)+solid lipid.(solid lipid. 12.7142)+liquid lipid.(liquid lipid.-2.285714286) ----eq 2
The Analysis of variance (ANOVA) (Table 2) gives information about effect of each factor, its interactions and the significant levels of these factors on the responses. Both the responses, particle size and drug entrapment efficacy were significantly influenced by the factor solid and liquid lipid concentration.
In the optimised formulation, the prediction profiler showed 80% desirability for the formulation formulated at a 0:0 labelled solid lipid and liquid lipid ratio, with predicted particle size of 132 nm and entrapment efficiency of 88. Hence the nanoparticles were prepared using the lipid ratio predicted by the design and this optimised formulation was subjected to stability studies.
Particle size analysis and zeta potential
The particle size and PDI indicates the steadiness of the product. The minute increase in particle size of NLC stored at 40±2ºC / 75 ±5%RH can be attributed to the higher temperature which may have caused nucleation of the nano clusters and enhanced the crystal growth. The particles stored at room temperature had excellent repulsive forces between them, resulting in good particle separation, but the changes in zeta potential of the particles stored at 40±2ºC / 75±5% RH may be due to the above-mentioned fact of crystal growth and cluster formation at higher temperature.
Scanning electron microscopy
The NLC formulations appeared distinct, with an even surface for the one stored at 25±2°C/60±5% RH. Upon 6 months of storage at 40±2ºC / 75±5% RH, the NLCs exhibited a slightly uneven surface which could be due to the crystal growth.
Atomic force microscopy
AFM has the competence to characterize nanoparticles in three dimensions with sub-nanometer precision. The separated lipid particles in the current sample showed a smooth or nearly smooth exterior even after 6 months of storage at 25 ±2ºC / 60±5% RH. However, after subjecting the samples to 40±2ºC / 75±5% RH, few agglomerations were observed correlating with the outcomes of particle size analysis and SEM.
Drug content
Although the NLC formulation contained solid and liquid lipid giving it a disordered inner core and making them less leaky and more rigid, loss of drug due to leakage had occurred during the stability studies. Least drug seepage was observed at 25ºC ±2ºC / 60% RH ± 5% RH. This may be due to a phase transition of the lipid during storage at a higher temperature, causing nanoparticle leakage.
In-vitro drug release studies
The initial release rate was compared to the release profile of nanostructured lipid carrier held at ambient temperature and under accelerated settings for six months. During the storage period, the reduction in the amount of the drug released may be because of increase in the size and leakage of drug due to higher temperature conditions.
Conclusion
The most important specifications of a nano-formulation are particle size, polydispersity, zeta potential, drug encapsulation efficiency, and surface morphology, which regulates the physical/chemical stability, release kinetics, and physical consistency of the product.
From the results, it is evident that there was no remarkable change in the parameters when stored at 25°C/60% RH for six months. Although the particle size and PDI increased after storing the particles at 40°C/75% RH for six months, the changes were not substantial as the particles were in the acceptable size range and PDI was quite low. The upsurge in particle size and PDI could be attributed to melting and further particle reaggregation at higher temperatures. Surface morphological study using SEM and AFM indicated that the nanoparticles were distinct, smooth and even when stored at 25°C/60% RH but slight changes were observed at elevated temperature and humidity.
The drug entrapment efficacy and drug release were found to be in acceptable range when kept at 25°C/60% RH. The entrapment efficiency of NLCs stored at 40°C/75% RH for six months showed a slight decrease with respect to the initial values. This may be attributed to the influence of heat, which might have led to a structural deformation and released the drug entrapped within the lipid matrix.
From the results, we can deduce that there was no appreciable variation found in the analysed specifications when stored at 25°C/60% RH. A very small variation was observed when stored at 40°C /75% RH which can be due to the accelerated environment. Thus, we can conclude that GB NLCs are stable and the recommended storage conditions for NLCs could be the room temperature.
Supporting File
References
1. Li Q, Cai T, Huang Y, Xia X, Cole SPC, Cai Y. A review of the structure, preparation, and application of NLCs, PNPs, and PLNs. Nanomaterials 2017; 7(6):1–25.
2. Muller RH, Radtke M, Wissing SA. Solid lipid nanoparticles (SLN) and nanostructured lipid carriers (NLC) in cosmetic and dermatological preparations. Adv Drug Deliv Rev 2002;54:131–55.
3. Hollander MH, Paarlberg KM, Huisjes AJM. Gestational Diabetes: A review of the current literature and guidelines. Obstet Gynecol Surv 2007;62(2):125–36.
4. Gupta S, Kesarla R, Chotai N, Misra A, Omri A. Systematic approach for the formulation and optimization of solid lipid nanoparticles of efavirenz by high pressure homogenization using design of experiments for brain targeting and enhanced bioavailability. Biomed Res Int 2017;3:1–18.
5. Yousef A, Dudhipala N, Majumdar S. Ciprofloxacin loaded nanostructured lipid carriers incorporated into in-situ gels to improve management of bacterial endophthalmitis. Pharmaceutics 2020;12(6):572.
6. Maroju RK, Barash S, Brisbane CE. Evaluation of a biologic formulation using customized design of experiment and novel multidimensional robustness diagrams. J Pharm Sci 2017;2:1–10.
7. Hommoss G, Pyo SM, Muller RH. Mucoadhesive tetrahydrocannabinol-loaded NLC- formulation optimization and long-term physicochemical stability. Eur J Pharm Biopharm 2017;117:408–17.
8. Poonia N, Kaur Narang J, Lather V, Beg S, Sharma T, Singh B, et al. Resveratrol loaded functionalized nanostructured lipid carriers for breast cancer targeting: Systematic development, characterization and pharmacokinetic evaluation. Colloids Surfaces B Biointerfaces 2019;181:756–66.
9. Aliasgharlou L, Ghanbarzadeh S, Azimi H, Zarrintan MH, Hamishehkar H. Nanostructured lipid carrier for topical application of n-acetyl glucosamine. Adv Pharm Bull 2016;6(4):581–587.
10. Saedi A, Rostamizadeh K, Parsa M, Dalali N, Ahmadi N. Preparation and characterization of nanostructured lipid carriers as drug delivery system: Influence of liquid lipid types on loading and cytotoxicity. Chem Phys Lipids [Internet]. 2018 Nov [cited 2018 Nov 25];216:65–72. Available from: http://www.ncbi.nlm.nih.gov/pubmed/30219661.
11. Du W, Li H, Tian B, Sai S, Gao Y, Lan T, et al. Biointerfaces development of nose to-brain delivery of ketoconazole by nanostructured lipid carriers against cryptococcal meningoencephalitis in mice. Colloids Surfaces B Biointerfaces 2019;183:110446
12. Injem VK, Sudheer P, Ashwini M. Formulation and evaluation of topical solid lipid nanoparticulate system of clobetasole propionate. Der Pharma Chem 2017;9(22):29–37.