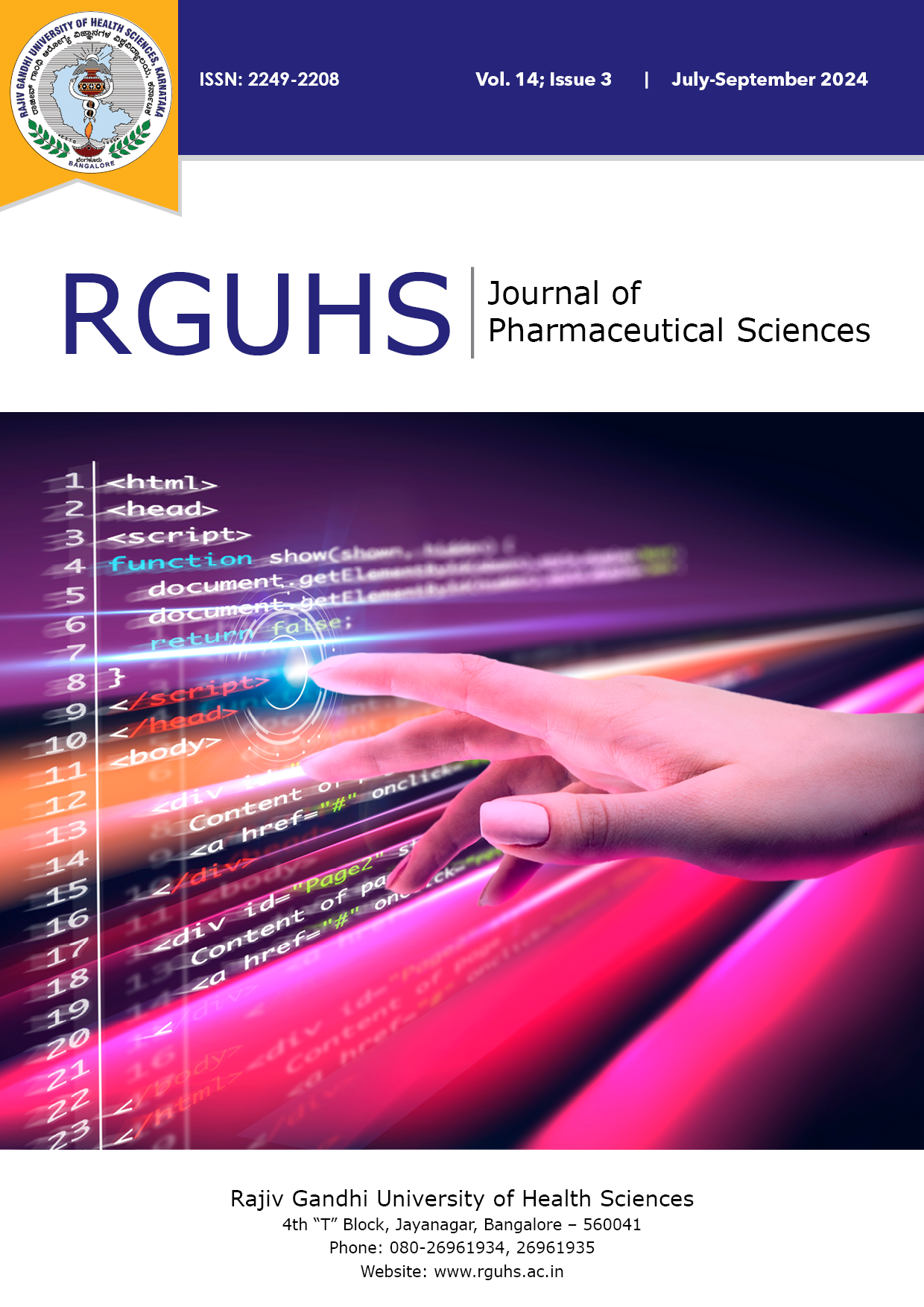
RJPS Vol No: 14 Issue No: 3 eISSN: pISSN:2249-2208
Dear Authors,
We invite you to watch this comprehensive video guide on the process of submitting your article online. This video will provide you with step-by-step instructions to ensure a smooth and successful submission.
Thank you for your attention and cooperation.
Suman Samaddar1*
*Corresponding author:
Suman Samaddar, Research Institute, BGS Global Institute of Medical Sciences, Kengeri, Bangalore – 560060 E-mail: sumanpppa@gmail.com Affiliated to Rajiv Gandhi University of Health Sciences, Bengaluru, Karnataka.

Abstract
Insulin-dependent diabetes is a multifactorial disorder characterized by β-cell dysfunction that results in metabolic failure. Isolated islets remain a lucrative area of diabetes research as these are miniature organ systems that are able to secrete insulin upon appropriate stimulation, without nervous control. This review encompasses the mechanisms responsible for beta cell destruction and regeneration from both beta and non-beta cells. Isolated islets are potential model for in vitro antidiabetic drug development, screening of antihyperglycemic agents and elucidating their mechanism of actions. In this review, various protocols of islet isolation have been discussed with their relative rates of success with regard to yield, purity, islet health, morphology and stability in culture conditions. Various islet protecting compounds of natural origin along with their mechanism of action have also been reviewed.
Keywords
Downloads
-
1FullTextPDF
Article
Introduction
Close to 350 million people worldwide suffers from diabetes in the recent times, out of which about 5% of patients suffer with Type 1 diabetes resulting from absolute loss of insulin production, and ~95% of patients live with Type 2 diabetes and impaired insulin sensitivity as well as with problematic insulin secretion. Islets of Langerhans, present within the pancreas, are miniature organ systems that are responsible mainly for the production of glucagon, insulin, somatostatin and pancreatic polypeptide post appropriate stimulation. Islet research is mainly aimed at curing and/or improving diabetes mellitus which results from a loss of insulin secretion from beta cells or reduced insulin sensitivity of tissues. Since their discovery in 1869, islets have been considered a potential in vitro system for a syndrome that cannot be imitated in a very effective manner using cell lines.1 Islets are miniature organ systems, preserving their architecture, differentiated status and insulin secretion capacity upon stimulation which is independent of nervous control. Islet isolation has fostered studies on the understanding of the pathophysiology of type I and II diabetes, transplantation and screening of hypoglycemic drugs.2,3 This review seeks to take an overview of the role of isolated islets as an experimental model in diabetes research.
Role of islets in glucose homeostasis
Langerhans islets are small island-like structures in the exocrine pancreas that represent only 1-2% of the total organ. Five different types of cells contribute to the release of hormones from the islets - α-cells (1520%) secrete glucagon; β-cells (65-80%) produce amylin, C-peptide and insulin; γ-cells (3-5%) secrete pancreatic polypeptide (PP); δ-cells (3-10%) produce somatostatin, and ε-cells (<1%) producing ghrelin.4-6 These hormones, altogether regulate the homoeostasis of glucose in vertebrates. Glucagon increases blood glucose level while insulin reduces it. Somatostatin prevents both glucagon and insulin release, while PP controls the activity of exocrine and endocrine secretion of the pancreas.7-9 Although the cellular composition of islets is similar within various species, i.e., humans, rats and mice, their cytoarchitecture differs considerably. While islets of rodents consist predominantly of central β cells and other types of cells in the periphery, human islets are composed of interconnected α- and β-cells.4,10 The pancreas, through insulin and glucagon, maintains blood glucose levels in a very close range of 4-6 mM. This conservation is achieved through the opposite and balanced actions of glucagon and insulin, known as glucose homeostasis. When blood glucose levels are low during sleep or in between meals, α-cells release glucagon to promote hepatic glycogenolysis. Furthermore, during extended fasting, glucagon stimulates hepatic and renal gluconeogenesis, which raises endogenous blood glucose levels.11 Insulin secretion from β-cells, on the other hand, is triggered by elevated exogenous glucose levels, such as those found after a meal.12 Insulin initiates the insulin-dependent absorption of glucose into muscle and adipose tissue after binding to its receptor on these tissues, lowering blood glucose levels by eliminating exogenous glucose from the bloodstream.13-15 Insulin also promotes glycogenesis,16-18 lipogenesis,19,20 and the incorporation of amino acids into proteins,21 making it an anabolic hormone as opposed to glucagon’s catabolic function.
Islet destruction in diabetes mellitus (DM) and regeneration
Islet destruction: β-cell death in diabetes mellitus
Type 1 and type 2 diabetes are the two most common types of diabetes characterized by progressive β-cell failure. This is usually triggered by an autoimmune attack on the β-cells, which results in progressive β-cell death in type 1 diabetes. Type 2 diabetes (T2DM) has a more varied pathogenesis, with varying degrees of β-cell failure along with varying degrees of insulin resistance. At the time of diagnosis, β-cell mass in type 1 diabetes is reduced by 70-80%. Because of the varying degrees of insulitis and the lack of visible β-cell necrosis, it was hypothesized that β-cell loss happens gradually over time.22 Initial pathological studies in type 2 diabetes people revealed a 25-50 percent loss of β-cells.23 Apoptosis of beta cells could thus be a common trait of both type 1 and type 2 diabetes. According to recent research, both types of diabetes are characterized by intra-islet production of inflammatory mediators (particularly the cytokine IL-1β), which leads to β-cell death, increasing β-cell loss, and diabetes.24,25 Invading immune cells create cytokines such as IL-1β, tumour necrosis factor (TNF)-α, and interferon (IFN)-γ in the insulitis lesion of type 1 diabetes. Interleukin-1β and/or TNF-α plus IFN-γ activate β-cell gene networks under the direction of the transcription factors NF-κB and STAT-1, resulting in apoptosis. NF-κB activation results in the generation of nitric oxide (NO) and chemokines, as well as calcium depletion in the endoplasmic reticulum (ER). Mitogen-activated protein kinases are activated, ER stress is triggered, and mitochondrial death signals are released, all of which contribute to β-cell death. In type 2 diabetes, chronic exposure to high amounts of glucose and free fatty acids (FFAs) induces β-cell dysfunction and may induce death. FFAs may cause β-cell apoptosis through ER stress, which is independent of NF-κB and NO. Thus, cytokines and nutrients cause β-cell death through fundamentally distinct pathways, with cytokines triggering an NF-κB-dependent mechanism that leads to caspase-3 activation and nutrients triggering an NF-κBindependent mechanism.26 De-differentiation of mature insulin-producing β-cells to a “naive” state has recently been identified as a novel mechanism of T2DM-related β-cell failure.27 As a result, the only way to improve insulin-dependent T2DM patients’ treatment is to replace or regenerate lost or defective β-cell mass. This could be accomplished by inducing β-cell proliferation or neogenesis, reversing β-cell dedifferentiation, or preventing -cell apoptosis.
Beta cell proliferation: Genes involved
Islet regeneration refers to the proliferation and replication of existing islet cells to enhance β-cell mass. In the prenatal and neonatal phases, pancreatic β-cells reproduce quickly. After these phases, however, the ability to replicate rapidly deteriorates. Furthermore, the ability to replicate in rodents and humans differs. Cell cycle regulators and circulating soluble substances regulate the proliferation of β-cells. Many mitogenic drugs have been proven to induce β-cell replication in young rats, but not in humans. Insulin-positive β-cells first arise during embryonic development in mice around embryonic day 13.5 and in humans around weeks 8-9. Beta cells are mostly produced during the fetal period by the differentiation of endocrine progenitor cells.28 Beta cells are produced by replicating existing β-cells during the late gestational and neonatal periods. After weaning, the rate of β-cell replication slows, and β-cell renewal capability is reduced until maturity or late adolescence.29,30 Multiple mitogenic signalling pathways, including IRS-PI3K-Akt, GSK3, mTOR, ChREBP/cMyc, Ras/Raf/Erk, and NFATs, are involved in beta cell replication. These methods also involve upstream activators of mitogenic signalling pathways, including nutrients (glucose, calcium), epidermal and platelet-derived GFs (Glp1, Gip), and hormones (leptin, estrogen, prolactin, and progesterone). Mitogenic signals affect the production of downstream cell cycle regulators such as cyclins, cyclin-dependent kinases (CDKs), cellcycle inhibitors, and E2F factors, causing quiescent cells to re-enter the cell cycle.31-36 Systemic regulators that affect β-cell proliferation during puberty, pregnancy, and obesity are circulating soluble substances coming from other organs. The modulation of β-cell proliferation in response to insulin resistance has been linked to a number of circulatory regulators. Intestinal peptides like GlP-1 and GIP-1,37,38 adipokines generated from adipose tissue like adipsin,39,40 resistin, and leptin,41,42 and skeletal-muscle released factors like Il-6 and Il-10 are all examples of circulating regulators.43,44 Whereas multiple mice studies45-47 have revealed that β-cells do not proliferate, lineage tracing investigations have confirmed that human β-cells proliferate and give birth to a progenitor/stem cell population. Reg (Regenerating islets derived proteins), HNF-6, SOx9, NeuroD1, Netrin-1 and Neurogenin-3 are among the genes and transcription factors involved in this process.48-52 Five REG proteins from the Reg gene family have been found in humans so far. In vivo investigations utilizing transgenic and knockout mice have demonstrated that several members of this family are involved in β-cell replication and/or neogenesis.53 In autoimmune type 1 diabetes, they also help to maintain β-cell mass.54
Transcription factors in β-cell proliferation
SOx-9, HNF-6, NGN-3, and NeuroD1 are among the transcription factors reported to be involved in the proliferation of β-cells. During pancreatic organogenesis, SOX9 is the first particular marker and maintenance factor of multipotential progenitors. SOx9 increases pluripotent progenitor cell proliferation and inhibits apoptosis in the embryonic pancreas. It regulates pancreatic progenitor cell survival via regulating Notch signalling.55 The homeodomain-containing transcrip- tion factor hepatocyte nuclear factor 6 (HNF-6) is an essential regulator of endocrine development. HNF6 is required for maintaining NGN-3 expression and appropriate pancreatic duct shape in the developing pancreas and has both early and late functions.56 NeuroD1, a downstream target of NGN-3, continues the endocrine differentiation procedure started by NGN3 and helps the mature islet cells maintain their differentiated phenotype.57 NGN-3 determines endocrine cell fate early in pancreatic endocrine development, while NeuroD1 drives endocrine cell differentiation.58
Transdifferentiation of pancreas
Islet neogenesis refers to the transdifferentiation of adult pancreatic stem cells, which are thought to be present in the ductal epithelium or acinar tissue, to increase β-cell bulk. The conversion of pancreatic alpha or delta cells into insulin-producing β-cells is known as transdifferentiation. This process is aided by a number of genes and proteins. INGAP, Gastrin, MAFA, PDX1, FOXA2, NKX2.2, NKX6.1, PAX4, and others are among them. Gastrin causes islet-cell neogenesis from pancreatic exocrine duct cells, MAfA reprograms differentiated pancreatic exocrine cells into cells that closely resemble β-cells, and INGAP initiates islet neogenesis. PDX-1 starts endocrine neogenesis, fOxA2 is a major upstream regulator of PDX-1, NKX2.2 regulates pancreatic endocrine differentiation, NKX6.1 keeps and expands the population of β-cell precursors as they progress from precursors to differentiated β-cells, and PAx4 is expressed in endocrine cell progenitors and directs the formation of beta and delta cells.59-66
Isolation of islets
for diabetic research, islet isolation from the pancreas of mice is a standard procedure. Collagenase perfusion, pancreatic digesting, and islet purification are the three processes of a traditional method. It is a critical step in achieving the necessary number and quality of islet separation. Because of its unique ability to produce digesting enzymes, the pancreas is very timesensitive when it comes to islet separation. As a result, decreasing variance during collagenase perfusion might improve the islet preparation process. Collagenase solution is perfused through the common bile duct. To put a needle into the common bile duct, direct puncture and catheterization through the gall bladder should be employed. Because of the size and length of the duct, performing such a puncture requires some experience, and it can be stressful, especially for inexperienced technicians.67
Islet purification
Purification of the islets is another important stage in the intended preparation. To purify islet from collagenasedigested pancreatic tissue, three procedures can be used: ficoll68-70 and iodixanol gradient separation,71 filtration,71-72 and magnetic retraction.73-74 A successful islet separation procedure includes enzyme-digesting the tissues connecting the islets to the exocrine tissue, isolating islets from non-islet tissue, and culturing isolated islets in an environment that favours cell viability. Because magnetic retraction is only suitable for islet isolation from humans and large animals, most researchers employ one of two methods to obtain islets from the pancreas of mice. The filtration procedure proposed by Salvalaggio et al.,67 and the Ficoll method introduced by Bluestone’s group are the two techniques.68 Mouse islet preparation was generated using the filtration procedure described by Salvalaggio et al., which included clamping the common bile duct, distending the pancreas with collagenase V, mincing the pancreas with surgical scissors, digesting and handshaking, and finally filtering through a 100 μm nylon cell strainer. This process resulted in an overall purity of 86 percent (before hand-shaking). The Ficoll approach has the drawback of perhaps being poisonous to the isolated islets. Additional cell straining with a 70 μm nylon cell strainer can be used to remove the exocrine cells and keep the bigger islets. Overall purity is estimated to be around 95%. The use of a density gradient to purify islets from acinar tissue is controversial. Because of the nature of pancreatic tissue, purifying islets from acinar tissue, regardless of the approach, is critical. Separation of islets from pancreatic acinar tissue is required because the exocrine pancreas cells emit numerous digesting enzymes. The hypertonic solution sodium diatrizoate, Histopaque (Sigma-Aldrich, St. Louis, MO, USA), is also employed to isolate different cell types. Our lab employed Histopaque 1077 to make the gradients, and a high level of purity was attained with only a few contaminated acinar cells, which were easily removed during the later handpicking of islets.2 The final purity of the product is determined by the animal strain and density gradient parameters. Lean rodents, in our experience, produce a purer final preparation than those with greater fat. The outcome of the gradient purification is also strain-dependent, which is consistent with data demonstrating strain-dependent changes in islet isolation.75 Prior to culture, a second purification of islets from acinar tissue is frequently required to further boost islet purity. Using a microscope, we detect the islets, then handpick them from one suspension culture dish and place them in a second dish containing a buffered solution or culture medium, such as HBSS or RPMI, respectively. Islets can then be transferred to a dish and cultured overnight in culture media.
Islet yield
The projected islet yield from an isolation method is highly dependent on the rodent’s age and strain. The total islet yield will be influenced by the technician’s expertise as well as the method of isolation employed. With the help of an experienced technician, yields from diverse mouse strains should range from 200 to 400 islets per mouse, with an average of 300 islets per mouse.3,76 Rats can produce between 600 and 800 islets per animal.75 In comparison, the human pancreas is thought to contain around one million islets, and islet equivalents estimate that a normal isolation provides approximately 250,000450,000 islets.77
Islet culture conditions
Islet isolation must be followed by suitable culture conditions to ensure that the islets can recover from the shock of collagenase digestion. Islets cultivated with 11 mM glucose have decreased apoptosis rates and improved vitality than those cultured in conditions with both higher and lower glucose concentrations for rodents.78 Extended exposure to high glucose produces toxicity, but media with glucose contents significantly below 11 mM can lower islet insulin content and down-regulate critical genes involved in glucose metabolism.78,79 RPMI 1640 with serum preserves or augments glucose-stimulated insulin secretion (GSIS) in murine islets, according to studies of optimal culture conditions.80 In order to avoid competition for nutrients, the recommended islet density for long-term culture is four islets/cm2.81 Islets have time to recover from the arduous process of collagenase digestion after an overnight incubation of 16-20 hours. Prior to completing viability or functional assessment experiments, islet function must be recovered in a sterile incubator at 370C with 5% CO2 infusion and humidified air.80 Rodent islets can maintain glucose sensitivity in culture for at least 1 week with regular medium changes. Changes in mouse islet function can occur in as short as 1 to 4 days in culture, according to studies from human islets80 and probably much longer.82,83
Islet health and morphology
A visual examination of the islets can provide some basic health information. A significant level of acinar or ductal tissue linked to the islets indicates an underdigested islet preparation. The islets in an overdigested islet preparation will have small ducts and few to no acinar cells, as well as rough edges (islets with rough edges may recover following an overnight culture). After an overnight culture in extreme cases of overdigestion, the islets will break into single cells. A normal, healthy islet will have smooth edges and be spherical. Islets appear round and golden brown when observed under a light microscope with a scanning objective lens, with a diameter of 50250 m. These characteristics, particularly the deeper hue of islets in comparison to the comparatively translucent exocrine tissue, makes it possible to identify islets quickly (Figure 1A-C).
Islet functionality: Glucose-stimulated insulin secretion (GSIS)
The ability of pancreatic islets to regulate the release of insulin and other hormones in direct reaction to variations in extracellular glucose concentration is one of their most important characteristics. Because only islet beta cells manufacture and release insulin in the body, this ability characterizes islet function in significant part. Glucosestimulated insulin secretion is thus a widely used indicator of islet function. Islets are cultivated in a ‘low’ glucose concentration, often about 3 mM, to measure the quantity of insulin produced into the media under ‘basal’ or ‘unstimulated’ conditions in order to determine GSIS. Insulin secretion is stimulated when islets are exposed to greater glucose concentrations, such as 11.1 mM (halfmaximal) or >28 mM (maximal). The islet response to glucose stimulation is biphasic, consisting of a fast spike in insulin release (first phase), followed by a slow decrease to a protracted second phase plateau of insulin that lasts for the length of the stimulus.84
Islet protecting agents
Isolated islands have been regularly used to investigate the potential of natural products to protect islets. A wide range of natural and synthetic substances have been utilized to screen for islet protecting and insulin secretagogue actions using cultured islets. The aqueous extract of Teucrium polium (Labiatae) was shown to have insulinotropic properties, as it increased insulin production by nearly 135% after a single dose in isolated islets cultivated under high glucose (16 mM).85 A significant increase in insulin secretion was seen in a perifusion system in which an exact number of Langerhans islets were exposed to different fractions of Urtica dioica extracts.86 Curcumin inhibited phosphorylation of inhibitor of kappa B alpha, which protected islets from cytokine-induced islet death in vitro by scavenging Reactive Oxygen Species (ROS) and normalising cytokine-induced NF-kB translocation (Iκβα). Streptozotocin (STZ) treated mice had higher levels of pro-inflammatory cytokine in their serum and pancreas, but not those pretreated with curcumin before STZ.87 Both glucose (8.3 mM) and glibenclamide (0.01 mM)-induced insulin secretion and phosphorylation of ERK1/2 were found to be potentiated by quercetin. Furthermore, quercetin protected β-cell activity and viability against oxidative damage caused by H2O2, as well as causing a substantial activation of ERK1/2.88 The effects of resveratrol and a possible relationship with SIRT1 in insulinoma INS-1E cells and human islets were examined using resveratrol-cultured insulinoma INS-1E cells and human islets. There was a significant potentiation of glucose-stimulated insulin secretion. This effect was linked to an increase in glycolytic flow, which resulted in more glucose oxidation, ATP production, and mitochondrial oxygen consumption. Upregulation of critical genes for β-cell function, such as GLUT2, glucokinase, PDX-1, HNF-1, and TFAM, was associated with these alterations. Sirt1 overexpression was also found in INS-1E cells after resveratrol administration.89 On streptozotocin-treated islets, the aqueous juice of the bitter gourd fruit Momordica charantia L. was evaluated. These changes were linked to upregulation of key genes for β-cell function, such as GLUT2, glucokinase, PDX-1, HNF-1, and TFAM. SIRT1 overexpression was also discovered in INS-1E cells following resveratrol treatment.89 The aqueous juice of the bitter gourd fruit Momordica charantia L. was tested on streptozotocintreated islets. It was discovered that it increased insulin production from STZ-islets, decreased lipid peroxidation, and decreased apoptosis, demonstrating its antioxidant and antiapoptotic properties.90 On Il1β induced pancreatic islets, the effects of quercetin and its metabolites quercetin 3′-sulfate, quercetin 3-glucuronide, and isorhamnetin 3-glucuronide were investigated. Quercetin suppressed IL-1β-induced IB phosphorylation, NFkB activation, and iNOS promoter activity, as well as reducing IL-1β-induced nitrite generation, iNOS protein, and iNOS mRNA expression. In addition, quercetin dramatically reduced the inhibitory effect of Il-1β on insulin secretion.91 GSIS was reported to be enhanced by an oleanane-type triterpenoid saponin derived from Momordica tuberosa (Cucurbitaceae), which reduced NO production, lipid peroxidation, and increased islet survival in STZ- and high glucose-treated islets.2
Conclusion
Isolated islets have established themselves as a popular model system in diabetes research. In contrast to complete organism systems, micro organ systems do not require neurological control and modification in terms of stimulation, secretion, transfection, and signalling pathways. This is also a powerful technique for in vitro screening of hypoglycemic drugs and understanding islet destruction and regeneration mechanisms. Although there are limitations to conclusive success, the improvements discussed here indicate that the future is bright.
Supporting File
References
- Dittrich HM, von Dorsche H. The anatomical and histological investigation of the pancreas in the 19th century and pancreas research from discovery of islets (1869) till the discovery of pancreas -diabetes 1889. AnatAnz 1978;143:231-41.
- Samaddar S, Koneri R, Varre JV, Jha DK. Triterpenoid saponin from Momordica tuberosa (Cucurbitaceae) stimulates insulin secretion from isolated mouse pancreatic islets and provides protection from streptozotocin and high-glucose induced injury. Indian J Pharm Educ Res 2019;53(2s):23-32.
- Shewade YM, Umrani M, Bhonde RR. Large-scale isolation of islets by tissue culture of adult mouse pancreas. Transplant Proc 1999;31:1721-3.
- Brissova M, Fowler MJ, Nicholson WE, Chu A, Hirshberg B, Harlan DM et al. Assessment of human pancreatic islet architecture and composition by laser scanning confocal microscopy. J Histochem Cytochem 2005;53:1087-97.
- Katsuura G, Asakawa A, Inui A. Roles of pancreatic polypeptide in regulation of food intake. Peptides 2002;23:323-29.
- Wierup N, Svensson H, Mulder H, Sundler F. The ghrelin cell: a novel developmentally regulated islet cell in the human pancreas. Regul Pept 2002;107:639.
- Goke B. Islet cell function: alpha and beta cells– partners towards normoglycaemia. Int J Clin Pract Suppl 2008;159:2-7.
- Hauge-Evans AC, King AJ, Carmignac D, Richardson CC, Robinson IC, Low MJ et al. Somatostatin secreted by islet delta-cells fulfills multipleroles as a paracrine regulator of islet function. Diabetes 2009;58:403-11.
- Batterham RL, Le Roux CW, Cohen MA, Park AJ, Ellis SM, Patterson M et al. Pancreatic polypeptide reduces appetite and food intake in humans. J Clin Endocrinol Metab 2003;88:3989-92.
- Cabrera O, Berman DM, Kenyon NS, Ricordi C, Berggren PO, Caicedo A. The unique cytoarchitecture of human pancreatic islets has implications for islet cell function. Proc Natl Acad Sci USA 2006;103:2334-39.
- Freychet L, Rizkalla SW, Desplanque N, Basdevant A, Zirinis P, Tchobroutsky G et al., Effect of intranasal glucagon on blood glucose levels in healthy subjects and hypoglycaemic patients with insulin-dependent diabetes. Lancet 1988;1:1364-66.
- Komatsu M, Takei M, Ishii H, Sato Y. Glucosestimulated insulin secretion: a newer perspective. J Diabetes Investig 2013;4:511-16.
- Khan AH, Pessin JE. Insulin regulation of glucose uptake: a complex interplay of intracellular signalling pathways. Diabetologia 2002;45:1475-83.
- Kohn AD, Summers SA, Birnbaum MJ, Roth RA. Expression of a constitutively active Akt Ser/Thr kinase in 3T3-L1 adipocytes stimulates glucose uptake and glucose transporter 4 translocation. J Biol Chem 1996;271:31372-78.
- Zisman A, Peroni OD, Abel ED, Michael MD, Mauvais-Jarvis F, Lowell BB et al. Targeted disruption of the glucose transporter 4 selectively in muscle causes insulin resistance and glucose intolerance. Nat Med 2000;6:924-8.
- Sibrowski W, Seitz HJ. Rapid action of insulin and cyclic AMP in the regulation of functional messenger RNA coding for glucokinase in rat liver. J Biol Chem 1984;259: 343-6.
- Kim SY, Kim HI, Kim TH, Im SS, Park SK, Lee IK et al. SREBP-1c mediates the insulin-dependent hepatic glucokinase expression. J Biol Chem 2004;279:30823-29.
- Aiston S, Hampson LJ, Arden C, Iynedjian PB, Agius L. The role of protein kinase B/Akt in insulin-induced inactivation of phosphorylase in rat hepatocytes. Diabetologia 2006;49:174-82.
- Walton PE, Etherton TD. Stimulation of lipogenesis by insulin in swine adipose tissue: antagonism by porcine growth hormone. J Anim Sci 1986;62:1584- 95.
- McTernan PG, Harte AL, Anderson LA, Green A, Smith SA, Holder JC et al. Insulin and rosiglitazone regulation of lipolysis and lipogenesis in human adipose tissue in vitro. Diabetes 2002;51:1493-98.
- Biolo G, Declan Fleming RY, Wolfe RR. Physiologic hyperinsulinemia stimulates protein synthesis and enhances transport of selected amino acids in human skeletal muscle. J Clin Invest. 1995;95:811-19.
- Kloppel G, Lo¨hr M, Habich K, Oberholzer M, Heitz PU. Islet pathology and the pathogenesis of type 1 and type 2 diabetes mellitus revisited. Surv Synth Pathol Res 1985;4:110-25.
- Clark A, Wells CA, Buley ID, Cruickshank JK, Vanhegan RI, Matthews DR, et al. Islet amyloid, increased α-cells, reduced β-cells and exocrine fibrosis: quantitative changes in the pancreas in type 2 diabetes. Diabetes Res 1988;9:151-9.
- Donath MY, Halban PA. Decreased β-cell mass in diabetes: significance, mechanisms and therapeutic implications. Diabetologia 2004;47:581-9.
- Donath MY, Storling J, Maedler K, MandrupPoulsen T. Inflammatory mediators and islet β-cell failure: a link between type 1 and type 2 diabetes. J Mol Med 2003;81:455-70.
- Cnop M, Welsh N, Jonas JC, Jorns A, Lenzen S, Eizirik DL. Mechanisms of pancreatic β-cell death in type 1 and type 2 diabetes-many differences, few similarities. Diabetes 2005;54 (Suppl 2):S97-S107.
- Granot Z, Swisa A, Magenheim J, Stolovich-Rain M, Fujimoto W, Manduchi E, et al. LKB1 regulates pancreatic beta cell size, polarity, and function. Cell Metab 2009;10(4):296-308.
- Finegood DT, Scaglia L, Bonner-Weir S. Dynamics of beta-cell mass in the growing rat pancreas. Estimation with a simple mathematical model. Diabetes1995;44:249-56.
- Dor Y, Brown J, Martinez OI, Melton DA. Adult pancreatic beta-cells are formed by self-duplication rather than stem-cell differentiation. Nature 2004;429:41-6.
- Meier JJ, Butler AE, Saisho Y, MonchampT, Galasso R, Bhushan A, et al. Beta-cell replication is the primary mechanism subserving the postnatal expansion of beta-cell mass in humans. Diabetes 2008;57:1584-94.
- Kulkarni RN, Mizrachi EB, Ocana AG, Stewart AF. Human beta-cell proliferation and intracellular signaling: driving in the dark without a road map. Diabetes 2012;61:2205-13.
- Bernal-Mizrachi E, Kulkarni RN, Scott DK, Mauvais-Jarvis F, Stewart AF, Garcia-Ocana A. Human beta-cell proliferation and intracellular signaling part 2: still driving in the dark without a road map. Diabetes 2014;63:819-31.
- Stewart AF, Hussain MA, Garcia-Ocana A, Vasavada RC, Bhushan A, Bernal-Mizrachi E, et al. Human beta-cell proliferation and intracellular signaling: part 3. Diabetes 2015;64:1872-85.
- . Rieck S, Zhang J, Li Z, Liu C, Naji A, Takane KK, et al. Overexpression of hepatocyte nuclear factor4alpha initiates cell cycle entry, but is not sufficient to promote beta-cell expansion in human islets. Mol Endocrinol 2012;26:1590-602.
- Cozar-Castellano I, Fiaschi-Taesch N, Bigatel TA, Takane KK, Garcia-Ocana A, Vasavada R, et al. Molecular control of cell cycle progression in the pancreatic beta-cell. Endocr Rev 2006;27:356-70.
- Huang C, Snider F, Cross JC. Prolactin receptor is required for normal glucose homeostasis and modulation of beta-cell mass during pregnancy. Endocrinology 2009;150:1618-26.
- Fusco J, Xiao X, Prasadan K, Sheng Q, Chen C, Ming YC, et al. GLP-1/Exendin-4 induces betacell proliferation via the epidermal growth factor receptor. Sci Rep 2017;7:9100-6.
- Buteau J, Foisy S, Joly E, Prentki M. Glucagon-like peptide 1 induces pancreatic beta-cell proliferation via transactivation of the epidermal growth factor receptor. Diabetes 2003;52:124-32.
- Lo JC, Ljubicic S, Leibiger B, Kern M, Leibiger IB, Moede T, et al. Adipsin is an adipokine that improves beta cell function in diabetes. Cell 2014;158:41-53.
- Retnakaran R. Adiponectin and beta-cell adaptation in pregnancy. Diabetes 2017;66:1121-2.
- Pham MN, Kolb H, Mandrup-Poulsen T, Battelino T, Ludvigsson J, Pozzilli P, et al. Serum adipokines as biomarkers of beta-cell function in patients with type 1 diabetes: positive association with leptin and resistin and negative association with adiponectin. Diabetes Metab Res Rev 2013;29:166-70.
- Park S, Hong SM, Sung SR, Jung HK. Long-term effects of central leptin and resistin on body weight, insulin resistance, and beta-cell function and mass by the modulation of hypothalamic leptin and insulin signaling. Endocrinology 2008;149:445-54.
- Dirice E, Kahraman S, Jiang W, El Ouaamari A, De Jesus DF, Teo AK, et al. Soluble factors secreted by T cells promote beta-cell proliferation. Diabetes 2014;63:188-202.
- Dauriz M, Trombetta M, Boselli L, Santi L, Brangani C, Pichiri I, et al. Interleukin-6 as a potential positive modulator of human beta cellfunction: an exploratory analysis-the Verona Newly Diagnosed Type 2 Diabetes Study (VNDS) 6. Acta Diabetol 2016;53:393-402.
- Chase LG, Ulloa-Montoya F, Kidder BL, Verfaillie CM. Islet-derived fibroblast-like cells are not derived via epithelial-mesenchymal transition from Pdx-1 or Insulin-Positive cells. Diabetes 2007;56:3-7.
- Atouf F, Park CH, Pechhold K, Malancha Ta, Choi Y, Lumelsky NL. No evidence for mouse pancreatic β-cell epithelial-mesenchymal transition in vitro. Diabetes 2007;56:699-702.
- Morton RA, Geras-Raaka E, Wilson LM, Raaka BM, Gershengorn MC. Endocrine precursor cells from mouse islets are not generated by epithelial-tomesenchymal transition of mature β-cells. Mol Cell Endocrinol 2007;270:87-93.
- Joglekar MC, Hardikar AA. Epithelial-tomesenchymal transition in pancreatic islet β-cells. Cell Cycle 2010;9:4077-9.
- Joglekar MV, Joglekar VM, Joglekar VM, Hardikarc AA. Human fetal pancreatic insulin-producing cells proliferate I. J Endocrinol 2009;201:27-36.
- Joglekar MV, Patil D, Joglekar VM, Rao GV, Reddy DN, Mitnala S, et al. The miR-30 family microRNAs confer epithelial phenotype to human pancreatic cells. Islets 2009;1:137-47.
- . Russ HA, Ravassard P, Kerr-Conte J, Pattou F, Efrat S. Epithelial-mesenchymal transition in cells expanded in vitro from lineage-traced adult human pancreatic β-cells. Plos One 2009;4:1-8.
- Bar Y, Russ HA, Knoller S, Ouziel-Yahalom L, Efrat S. HES-1 is involved in adaptation of adult human β-cells to proliferation in vitro. Diabetes 2008;57:2413-20.
- Liu J, Cui W, Li B, Lu Y. Possible roles of Reg family of proteins in pancreatic islet cell growth. Endocr Metab Immune Disord Drug Targets 2008;8:1-10.
- Singh B, Nikoopour E, Huszarik K, Elliott JF, Jevnikar AM. Immunomodulation and regeneration of islet β-cells by cytokines in autoimmune type 1 diabetes. J Interferon Cytokine Res 2011 31:711-9.
- Seymour PA, Freude KK, Tran MN, Mayes EE, Jensen J, Kist R, et al. SOX9 is required for maintenance of the pancreatic progenitor cell pool Proc Natl Acad Sci USA 2006;104: 1865-70.
- Zhang H, Ables ET, Pope CF, Washington MK, Hipkens S, Means AL, et al. Multiple, temporalspecific roles for HNF6 in pancreatic endocrine and ductal differentiation. Mech Dev 2009;126:958-73.
- Jensen J. Gene regulatory factors in pancreatic development. Devel Dyna 2004;229:176-200.
- Gasa R, Mrejen C, Lynn FC, Cox P, Sanchez L, Yang KY, et al. Induction of pancreatic islet cell differentiation by the neurogenin-neuroD cascade. Differentiation 2008;76:381-91.
- Pittenger GL, Taylor-Fishwick D, Vinik AI. The role of islet. neogeneis-associated protein (INGAP) in pancreatic islet neogenesis. Curr Protein Pept Sci 2009;10:37-45.
- Rooman I, Lardon J, Bouwens L. Gastrin stimulates β-cell neogenesis and increases islet mass from transdifferentiated but not from normal exocrine pancreas tissue. Diabetes 2002;51:686-90.
- Zhou Q, Brown J, Kanarek A, Rajagopal J, Melton DA. In vivo reprogramming of adult pancreatic exocrine cells to β-cells. Nature 2008;455:627-33.
- Taniguchi H, Yamato E, Tashiro F, Ikegami H, Ogihara T, Miyazaki J. β-cell neogenesis induced by adenovirus-mediated gene delivery of transcription factor pdx-1 into mouse pancreas. Gene Ther 2003;10:15-23.
- Lee CS, Sund NJ, Vatamaniuk MZ, Matschinsky FM, Stoffers DA, Kaestner KH. Foxa2 controls Pdx1 gene expression in pancreatic β-cells in vivo. Diabetes 2002;51:2546-51.
- Hill JT, Chao CS, Anderson KR, Kaufman F, Johnson CW, Sussel L. Nkx2.2 activates the ghrelin promoter in pancreatic islet cells. Mol Endocrinol 2010;24:381-90.
- . Sander M, Sussel L, Conners J, Scheel D, Kalamaras J, Cruz FD, et al. Homeobox gene Nkx6.1 lies downstream of Nkx2.2 in the major pathway of β-cell formation in the pancreas. Development 2000;127:5533-40.
- Sosa-Pineda B, Chowdry K, Torres M, Oliver G, Gruss P. The Pax-4 gene is essential for differentiation of insulin-producing β-cells in mammalian pancreas. Nature 1997;386:399-402.
- Salvalaggio PRO, Deng S, Ariyan CE, Millet I, Zawalich WS, Basadonna GP, Rothstein DM. Islet filtration: a simple and rapid new purification procedure that avoids ficoll and improves islet mass and function. Transplantation 2002;74:877-9.
- Szot GL, Koudria P, Bluestone JA. Murine pancreatic islet isolation. J Vis Exp 2007;255:1-4.
- Shapiro AM, Lakey JR, Ryan EA, Korbutt GS, Toth E, Warnock GL, Kneteman NM, Rajotte RV. Islet transplantation in seven patients with type 1diabetes mellitus using a glucocorticoidfree immunosuppressive regimen. N Engl J Med 2000;343:230-8.
- Warnock GL, Meloche RM, Thompson D, Shapiro RJ, Fung M, Ao Z. Improved human pancreatic islet isolation fora prospective cohort study of islet transplantation vs best medical therapy in type 1 diabetes mellitus. Arch Surg 2005;140:735-44.
- Delle H, Saito MH, Yoshimoto PM, Noronha IL. The use of iodixanol for the purification of rat pancreatic islets. Transplant Proc 2007;39:467-9.
- Yonekawa Y, Matsumoto S, Okitsu T, Arata T, Iwanaga Y, Noguchi H. Effective islet isolation method with extremely high islet yields from adult pigs. Cell Transplant 2005;14:757-62.
- Tons HA, Baranski AG, Terpstra OT, Bouwman E. Isolation of the islets of Langerhans from the human pancreas with magnetic retraction. Transplant Proc 2008;40:413-4.
- Banerjee M, Otonkoski T. A simple two-step protocol for the purification of human pancreatic beta cells. Diabetologia 2009;52:621-5.
- de Groot M, de Haan BJ, Keizer PP, Schuurs TA, van Schilfgaarde R, LeuveninkHG. Rat islet isolation yield and function are donor strain dependent. Lab Anim 2004;38(2):200-6.
- Gotoh M, Maki T, Kiyoizumi T, Satomi S, Monaco AP. An improved method for isolation of mouse pancreatic islets. Transplantation 1985;40(4):437-8.
- Street CN, Lakey JR, Shapiro AM, Imes S, Rajotte RV, Ryan EA et al. Islet graft assessment in the Edmonton protocol: implications for predicting longterm clinical outcome. Diabetes 2004;53(12):3107- 14.
- Efanova IB, Zaitsev SV, Zhivotovsky B, Kohler M, Efendic S, Orrenius S et al. Glucose and tolbutamide induce apoptosis in pancreatic beta-cells. A process dependent on intracellular Ca2+ concentration. J Biol Chem 1998;273(50):33501-7.
- Robertson RP, Harmon J, Tran PO, Tanaka Y, Takahashi H. Glucose toxicity in beta-cells: type 2 diabetes, good radicals gone bad, and the glutathione connection. Diabetes 2003;52(3):581-7.
- Andersson A. Isolated mouse pancreatic islets in culture: effects of serum and different culture media on the insulin production of the islets. Diabetologia 1978;14(6):397-404.
- de Haan BJ, Faas MM, Spijker H, vanWilligen JW, deHaan A, deVos P. Factors influencing isolation of functional pancreatic rat islets. Pancreas 2004;29(1):e15-e22.
- Gaber AO, Fraga D. Advances in long-term islet culture: the Memphis experience. Cell Biochem Biophys 2004;40(3 Suppl):49-54.
- Gilon P, Jonas JC, Henquin JC. Culture duration and conditions affect the oscillations of cytoplasmic calcium concentration induced by glucose in mouse pancreatic islets. Diabetologia 1994;37(10):1007- 14.
- Nolan AL, O’Dowd JF. The measurement of insulin secretion from isolated rodent islets of langerhans. Methods Mol Biol 2009;560:43-51.
- Esmaeili MA, Yazdanparast R. Hypoglycaemic effect of Teucrium polium: studies with rat pancreatic islets. J Ethnopharmacol 2004;95:27-30.
- Farzami B, Ahmadvand D, Vardasbi S, Majin FJ, Khaghani SH. Induction of insulin secretion by a component of Urtica dioica leave extract in perifused Islets of Langerhans and its in vivo effects in normal and streptozotocin diabetic rats. J Ethnopharmacol 2003;89:47-53.
- Kanitkar M, Gokhale K, Galande S, Bhonde RR. Novel role of curcumin in the prevention of cytokineinduced islet death in vitro and diabetogenesis in vivo. British J Pharmacol 2008;155:702-13.
- Youl E, Bardy G, Magous R, Cros G, Sejalon F, Virsolvy A. Quercetin potentiates insulin secretion and protects INS-1 pancreatic β-cells against oxidative damage via the ERK1/2 pathway. British J Pharmacol 2010;161:799-814.
- Vetterli L, Brun T, Giovannoni L, Bosco D, Maechler P. Resveratrol potentiates glucose-stimulated insulin secretion in INS-1E β-cells and human islets through a SIRT1-dependent mechanism. J Biol Chem 286;2011(8):6049-60.
- Sitasawad SL, Shewade Y, Bhonde R. Role of bitter gourd fruit juice in STZ-induced diabetic state in vivo and in vitro. J Ethnopharmacol 2000;73:71-9.
- Choa JM, Changa SY, Kimb DB, Needsc PW, Joa YH, Kima MJ. Effects of physiological quercetin metabolites on interleukin-1β-induced inducible NOS expression. J Nutriti Biochemy 2012;23:1394- 1402.