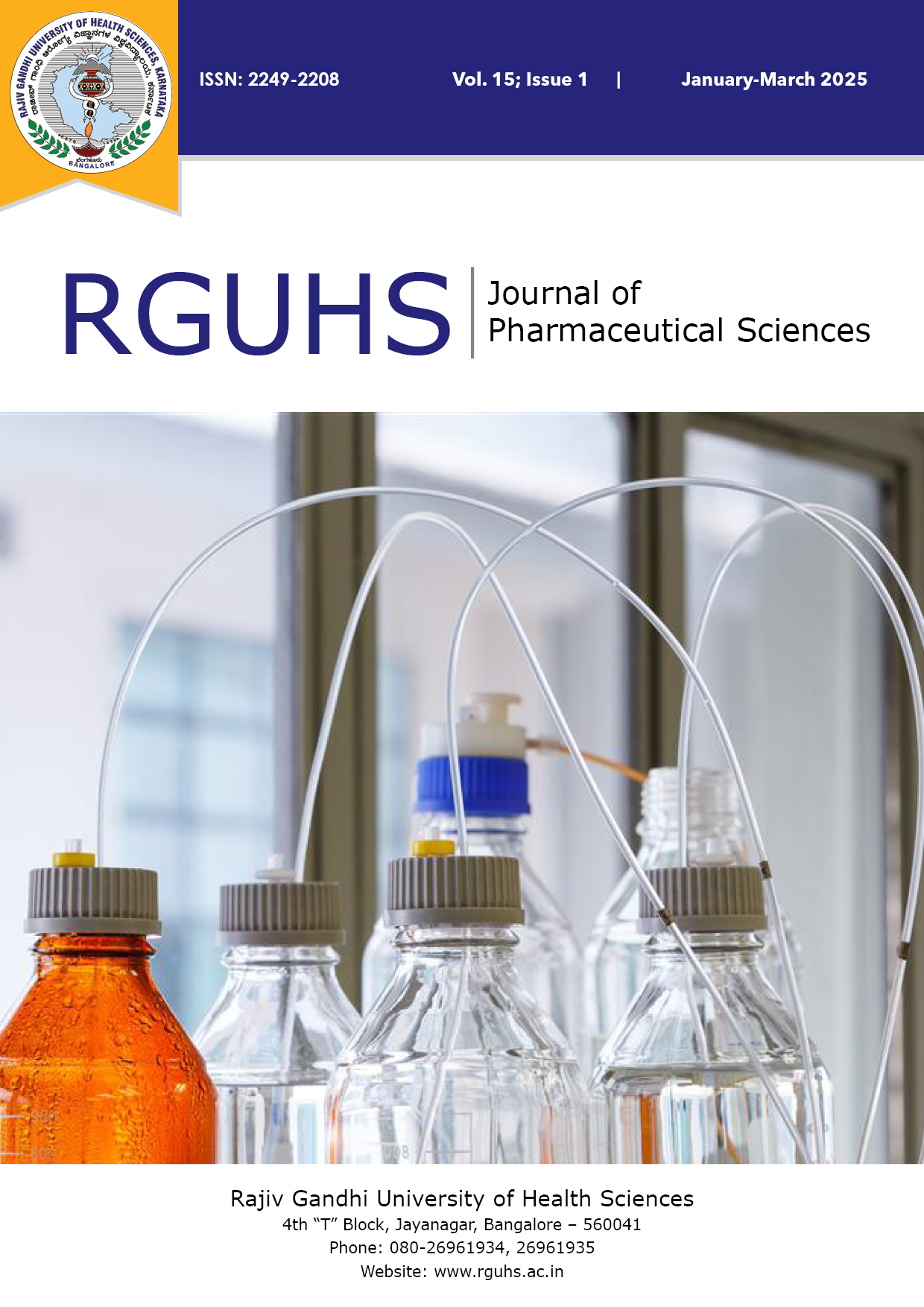
Abbreviation: RJPS Vol No: 15 Issue No: 1 eISSN: pISSN:2249-2208
Dear Authors,
We invite you to watch this comprehensive video guide on the process of submitting your article online. This video will provide you with step-by-step instructions to ensure a smooth and successful submission.
Thank you for your attention and cooperation.
1Dr. G R Godge, Associate Professor, Department of Pharmaceutics, Dr. Vithalrao Vikhe Patil Foundation’s College of Pharmacy, Ahmednagar, Maharashtra, India.
2Department of Pharmaceutics, Dr. Vithalrao Vikhe Patil Foundation’s College of Pharmacy, Ahmednagar, Maharashtra, India.
3Department of Pharmaceutics, Dr. Vithalrao Vikhe Patil Foundation’s College of Pharmacy, Ahmednagar, Maharashtra, India.
4Department of Pharmaceutics, Dr. Vithalrao Vikhe Patil Foundation’s College of Pharmacy, Ahmednagar, Maharashtra, India.
5Department of Pharmaceutics, Dr. Vithalrao Vikhe Patil Foundation’s College of Pharmacy, Ahmednagar, Maharashtra, India.
6PRES’s Pravara Rural College of Pharmacy (Diploma), Pravaranagar, Loni, Maharashtra, India.
*Corresponding Author:
Dr. G R Godge, Associate Professor, Department of Pharmaceutics, Dr. Vithalrao Vikhe Patil Foundation’s College of Pharmacy, Ahmednagar, Maharashtra, India., Email: grgodge@yahoo.com
Abstract
In a variety of fields, including clinical medicine, cancer therapies, and drug delivery, solid lipid nanoparticles (SLNs) are essential. Solid lipid, emulsifier, and water/solvent comprise SLNs. Triglycerides (tri-stearin), partial glycerides (Imwitor), fatty acids (stearic acid, palmitic acid), steroids (cholesterol), and waxes (cetyl palmitate) are examples of the lipids that can be utilized. Due to their many benefits, such as their capacity to increase the solubility of poorly soluble drugs, SLNs have garnered a lot of attention in the field of pharmaceutical administration. This increases the medications' bioavailability and therapeutic effectiveness. Additionally, by allowing controlled and extended drug release, SLNs facilitate precision dosage and decreased administration frequency. They serve as support vehicles as well as stabilizers, solubilizers, enhancers of permeation, transfection agents, and release rate modifiers. Controlled drug release systems can be developed with the use of SLNs, which are digestible drug delivery systems. For oral administration applications, a fast/slow-release drug delivery system could be achieved by adjusting the digestion rate of SLNs. One of the key advantages of lipid nanoparticles, especially SLNs, is their safety and biocompatibility. Lipid-based formulations are less likely to have adverse effects because the body typically tolerates lipids well. They are suitable for use in pharmaceutical applications because they can transport a range of therapeutic substances, including small medicine molecules and biotechnology materials. Lipid nanoparticle optimization is still challenging despite its potential. Researchers are always trying to make their formulations better to ensure the safety, efficacy, and scalability of their work.
Keywords
Downloads
-
1FullTextPDF
Article
Introduction
With the profound understanding achieved in various domains of biotechnology, biomedical engineering and nanotechnology, the field of novel drug delivery systems is growing at an exponential rate.1 The creation of nanoscale structures carrying the active pharmaceutical ingredients (API) is a common application of nanotechnology in several contemporary formulation techniques.2 The National Nanotechnology Initiative (NNI) defines nanotechnology as the study and application of structures that are generally between one and one hundred nanometers in size. The ultimate objective of nanotechnology is the same as that of medicine; to use targeted and controlled medication delivery to treat patients as effectively and side-effect-free as feasible, and to diagnose as precisely and early as possible.3 Using the principles of nanotechnology, several significant drug delivery systems have been developed, including solid lipid nanoparticles, nanoparticles, nanosuspension, nanoemulsion, and nanocrystals.4 The primary subject of this article is solid lipid nanoparticles (SLNs).
SLNs, which were first commercialized in 1991, offer an improved and substitute colloidal carrier system to emulsions, liposomes, and polymeric micro and nanoparticles.5 The medications utilized in SLNs are from BCS Classes II and IV, and the system is a colloidal carrier made up of a solid core of a high melting point lipid coated with an aqueous surfactant.2 Solid lipid takes the place of liquid lipid in SLNs when compared to other colloidal carriers. From lipid pellets for oral drug administration, the use of solid lipid as a matrix material for drug delivery is widely recognized.6 In a broad sense, triglycerides, partial glycerides, fatty acids, hard fats, and waxes are all considered to be lipids. The fact that the lipid matrix in SLN is composed of physiological lipids, which lowers the risk of both acute and chronic toxicity, is a definite benefit.7 It has been demonstrated that using solid lipid rather than liquid lipid improves the stability of integrated chemically-sensitive lipophilic components and increases control over the release kinetics of encapsulated substances. Numerous physicochemical traits connected to the lipid phase's physical state are the cause of these possibly advantageous effects. Firstly, compared to a liquid matrix, a solid matrix has less reactive agent mobility, which lowers the pace of chemical breakdown processes. Second, it is possible to regulate the micro phase separations between the carrier lipid and active ingredients within individual liquid particles. This keeps active compounds from building up at the surface of lipid particles, where chemical degradation reactions frequently take place. Thirdly, it has been demonstrated that incorporating poorly absorbed bioactive chemicals into solid lipid nanoparticles increases their absorption. It has also been demonstrated by a number of studies that using a solid matrix rather than a liquid matrix can slow down lipid breakdown and permit a longer-lasting release of the encapsulated component. Aqueous surfactants are another important class of excipients used in SLNs. They primarily serve as stabilizers for the dispersion of SLNs and their choice, as well as emulsifiers to create o/w type emulsions. They typically consist of a solid hydrophobic core with the medication dissolved or distributed throughout.8
High pressure homogenization or micro emulsification are the primary methods used to prepare SLNs. SLNs made using any method are in the dispersion form, which causes instability when stored for an extended period due to hydrolysis reactions. To improve their stability, lyophilisation is a cheap and simple alternative to lyophilisation that turns them into solid, dry, reconstitutable powders.9 In general, solid lipid nanoparticles present a promising platform for drug administration because of their biocompatibility, capacity to achieve regulated release, and capability to efficiently target and protect pharmaceuticals.10
Methods of Preparation of Solid Lipid Nanoparticles
1) High-pressure homogenization
A method frequently employed to create solid lipid nanoparticles (SLNs) is high-pressure homogenization. It involves applying high pressure to move a liquid that contains medicines and lipids through a small opening, usually a few microns wide. The applied pressure can range from 100 to 2000 bars. The liquid experiences significant acceleration during highpressure homogenization, reaching speeds of more than 1000 km/h. The larger particles in the formulation are disrupted by these forces, which cause them to shrink to submicron sizes. The formulation's normal lipid content ranges from 5% to 10%, and this does not provide a difficulty for the high-pressure homogenizer. Overall, high-pressure homogenization is a dependable method for creating solid lipid nanoparticles because it enables particle size reduction and efficient drug encapsulation within lipid matrix.5-10
a) Hot homogenization technique
To create lipid-based formulations like solid lipid nanoparticles and nano-emulsions, hot homogenization is used. It includes creating a pre-emulsion at temperatures over the melting point of lipids, which is then processed further using high-pressure homogenizers. The creation of a pre-emulsion is the first stage in hot homogenization. A drug-loaded lipid melt and an aqueous surfactant solution are combined at high temperatures to achieve this. At temperatures above the lipid melting point, the pre-emulsion is passed through a piston gap homogenizer in this step. The sample is subjected to the high pressure, which normally ranges from 500 to 1500 bar. During this process, the sample's temperature may rise to about 100°C. The repeated cycles make sure that the lipid droplets are equally distributed throughout the formulation and further reduced in size.3,5,6,10
b) Cold homogenization technique
Similar to heat homogenization, cold homogenization starts with the medication being dissolved in molten lipids. The drug-containing melt is quickly cooled during cold homogenization, which encourages homogenous drug distribution inside the solid lipid matrix. Low-temperature use makes lipids and particle communication more brittle. Cold homogenization often produces samples with broader size distributions and bigger particle sizes than hot homogenization. However, the benefits of cold homogenization include better drug stability because of reduced exposure to high temperatures and better drug dispersion within the lipid matrix.3,5,6,10
2) Ultra-sonication or high-speed homogenization technique
Two frequently utilized techniques for SLN preparation are high-speed homogenization and ultra-sonication. Lipid materials are dispersed in a hot aqueous surfactant solution during high-speed homogenization, which is followed by high-speed mixing to crush the particles. On the other hand, ultra-sonication uses high-frequency sound waves to disintegrate the lipid particles and produce smaller particles. The preparation of SLNs using high-speed homogenization and ultrasonication is beneficial for improving the oral bioavailability of medicines that are poorly water-soluble.5,13
3) Microemulsion technique
The procedure starts with stirring a clear fluid between 65 and 700 degrees Celsius. A low-melting fatty acid, an emulsifier, co-emulsifiers, and water are the typical ingredients in this mixture. After being heated to temperatures between 2°C and 30°C, the hot microemulsion which has droplets in the micron range is then dispersed into cold water. The droplets in the hot microemulsion are further condensed into submicron-sized particles by dilution with cold water. The generated nanoparticles can be used in a variety of industries, including nanotechnology, cosmetics, and medication delivery. These methods are superior to the earlier methods for solubility enhancement viz. inclusion or cyclodextrin complex, solid dispersion technique, nanosuspension technology, and formulation strategies for low absorption window.14-23
4) Solvent emulsification - Evaporation technique
The hydrophobic drug and the lipophilic material (such as lipids or surfactants) are dissolved in a waterimmiscible organic solvent, such as cyclohexane, dichloromethane, chloroform, or toluene, in the solvent emulsification-evaporation process. For fine emulsification and smaller droplet sizes, this step is essential. After micro-fluidization, the organic solvent is forced out of the emulsion. These nanoparticles can be further described, employed for a variety of purposes, including the administration of drugs, and have a small particle size.5
5) Solvent emulsification - Diffusion technique
An approach for creating nanoparticles that contain a drug or lipid is the solvent emulsification diffusion method. To create the desired nanoparticle formulation, multiple stages are needed.
Selection of Solvent: The solvent chosen for this procedure must be soluble in water to some extent. Benzyl alcohol, butyl lactate, ethyl acetate, isopropyl acetate, and methyl acetate are a few typical examples. Water and Solvent Saturation: To achieve initial thermodynamic equilibrium between the two liquids, the solvent and water are mutually saturated before the operation begins. Emulsification: A solvent-saturated aqueous phase with a stabilizer is used to emulsify the organic phase, which consists of a drug and lipid dissolved in a water-saturated solvent. Emulsification is frequently aided by a mechanical stirrer. The stabilizer aids in avoiding coalescence and stabilizing the emulsion that results. Dilution: Water (a dilution medium) is introduced to the emulsion to allow solvent diffusion into the continuous phase and encourage the creation of nanoparticles. Depending on the required final qualities of the nanoparticles, the ratio of water introduced into the solution normally ranges from 1:5 to 1:10. Solvent Diffusion and Nanoparticle Formation: As more water is included into the emulsion, the solvent diffuses from the internal phase (organic phase) into the continuous phase (water). This process results in the formation of nanoparticles. After being defined, the nanoparticles can be put to use in a variety of medical and other applications, including medication administration.5,24-26
6) Adsorption onto solid carriers
This process entails coating solid carriers like calcium silicate, silicon dioxide, or magnesium aluminometasilicate with a liquid lipid formulation in order to absorb it. This method is quick and affordable. To achieve adsorption, the solid carriers and liquid lipid mixture are typically combined in a blender. The choice of the carrier is crucial because it should have great liquid formulation adsorption capabilities and show good flow characteristics following adsorption. By converting liquid formulations into solid forms, this technique enables increased stability, simpler handling, and perhaps even improved drug delivery qualities.5
7) Melt granulation
A meltable binder is used and the powder combination is combined with it. High-shear mixing creates friction which melts the binder. Small granules are created as a result of the melted binder creating liquid bridges between the powder particles. Lecithins, polyoxylglycerides, partial glycerides, and polysorbates are examples of lipid excipients that can be used in the melt granulation approach to create a self-micro-emulsifying system. Some benefits from melt granulation are improved flow characteristics, controlled release profiles, and increased formulation stability. Mostly various medicated chewing gums, pectinate beads, mouth dissolving technology, etc. utilizes this melt granulation technique.27-29
8) Melt extrusion/spheronisation
Extrusion is a manufacturing procedure frequently used to create products with a consistent shape and density out of raw materials with plastic qualities. It entails applying pressure while maintaining a controlled product flow and temperature. To achieve best product quality, excipients viz. polysorbate 80, lauroyl polyoxyl glycerides, and sucrose monopalmitate are used. In this, the matrix core contained Gelucire 44/14, and hypromellose was molded inside an ethyl cellulose pipe. The core formulation was shielded from mechanical and hydrodynamic stresses by this combination.
For medications with low dosages but high potencies and melt extrusion, a solvent-free process has become a key approach for attaining significant drug loading and content consistency. This method allows for the uniform distribution of a significant number of active medicinal components throughout the formulation viz. mucoadhesive technique, mouth dissolving technique, etc.30-31
9) Supercritical fluid-based methods
Lipids can be employed to coat medication particles or create solid dispersions in supercritical fluid-based techniques. The procedure entails distributing the drug particles in a supercritical fluid that also contains one or more coating materials that have been dissolved under extreme pressure and heat. This approach employs a number of procedures, including:
RESS (Rapid Expansion of Supercritical Solution):
In this procedure, the coating ingredients are dissolved in a supercritical fluid, and the solution is then rapidly expanded through a nozzle or aperture. The supercritical fluid experiences a phase shift as a result of the quick expansion, creating tiny particles that coat the drug particles.
In these processes, carbon dioxide (CO2) is frequently utilized as the supercritical fluid. It has many benefits, including being non-toxic, inflammable, and easily accessible. Additionally, CO2 is excellent for many medicinal applications since it has a comparatively low critical temperature and pressure. These lipid-based excipients can benefit the drug's solubility, bioavailability, and controlled release from coated particles, among other things. The selection of an excipient is based on the particular needs of the medication formulation and the desired properties.5-10
Models for incorporation of active compounds into SLNs
The three incorporation models for solid lipid nanoparticle (SLN) research are as follows:
a) A solid lipid and active component solution is used to create the SLN in the Type I or homogeneous matrix model. Within the lipid blend, the active component is molecularly distributed. The cold homogenization procedure is used to create the SLN, and a dependable result is attained. To ensure uniform dispersion of active molecules throughout the lipid nanoparticles and prevent any enrichment in certain areas, the lipid blend is solidified, and the resulting solid is crushed.
b) This model, known as a Type II or drug-enriched shell model, is created utilizing the hot homogenization approach. The melted lipid contains a low concentration of the active component. The lipid precipitates first during the cooling of the hot oil-in-water nanoemulsion, which causes an increase in the concentration of active molecules in the melt that is left over. The active component and lipid are both contained in the outer shell that develops and solidifies. A burst release of the active substance may result from this enrichment of the particle's outer region. The proportion of active chemicals concentrated in the outer shell can be changed in a controlled shell model.
c) The drug-enriched core model, also known as type III, is when the active component concentration in the lipid melt is high and somewhat close to its saturation solubility. The solubility of the active component in the melt reduces as the heated oil droplets cool. The active molecules precipitate when the saturation solubility is reached, creating a drug-enriched core inside the solid lipid nanoparticles. These simulations show how the active ingredient can be integrated into solid lipid nanoparticles in a variety of ways, depending on the lipid characteristics, surfactant concentration, and manufacturing process.32,33
Characterization of solid lipid nanoparticles
- Particle size and zeta potential: Dynamic light scattering (DLS), which analyses the intensity variations of scattered light brought on by the Brownian motion of particles, can be used to quantify particle size. On the other hand, zeta potential can be assessed using electrophoretic mobility methods and offers details regarding the surface charge of the particles.5,34-36
- Dynamic light scattering: DLS is a commonly used technique to measure the size distribution of nanoparticles in a suspension. By analyzing the intensity fluctuations of scattered light, DLS can provide information about the hydrodynamic diameter of SLNs.5,34-36
- Static light scattering/ Fraunhofer diffraction: This technique used to determine the particle size of SLNs based on the analysis of the intensity of light scattered by the particles. This method can provide information about the size distribution and mean particle size of SLNs.5,34-36
- Electron microscopy: Transmission electron microscopy (TEM) or scanning electron microscopy (SEM), can be used to visualize the morphology and structure of SLNs. These techniques provide high-resolution images and can reveal details about the particle shape, surface characteristics, and internal structure.5,34-36
- Atomic force microscopy (AFM): It is a technique used to characterize the surface topography of nanoparticles, including SLNs. AFM uses a sharp probe to scan the surface of the sample, providing information about the particle size, shape, and surface roughness at a nanoscale resolution.5,34-36
- Acoustic methods: Ultrasound spectroscopy or acoustic attenuation measurements can be used to analyze the physical properties of SLNs. These methods can provide information about the particle size, aggregation state, and stability of SLNs based on the analysis of sound waves interacting with the nanoparticles.5,34-36
- Nuclear magnetic resonance: NMR spectroscopy can be used to study the physical and chemical properties of SLNs. NMR can provide information about the lipid composition, crystallinity, and interactions within the nanoparticles. It can also be used to investigate the drug-loading capacity and release behavior of SLNs.5,34-36
- Differential scanning calorimetry: It is a thermal analysis technique used to characterize the thermal behaviour of SLNs. DSC measures the heat flow associated with changes in the sample's heat capacity, which can provide information about the phase transitions, melting behaviour, and thermal stability of SLNs.5,34-36
- Powder X-ray diffractometry (PXRD): It is a technique used to analyze the crystalline structure of SLNs. By measuring the diffraction pattern of X-rays scattered by the nanoparticles, PXRD can provide information about the crystallographic phases, degree of crystallinity, and polymorphic forms present in the SLNs.5,34-36
- Storage stability of SLN: Storage stability refers to the ability of SLNs to maintain their physicochemical properties over time. It can be assessed by monitoring changes in particle size, zeta potential, drug content, drug release behaviour, and physical stability (e.g., sedimentation, aggregation) of SLNs during storage under various conditions, such as temperature, humidity, and light exposure.5,34-36
- In-vitro and ex-vivo methods for assessing drug release from SLN: These are used to evaluate the release of drugs from SLNs. In-vitro methods involve studying drug release in simulated physiological conditions outside the body, such as using dissolution testers or dialysis membranes. Ex-vivo methods, on the other hand, involve using tissue or organ samples to simulate drug release behaviour in a more physiological context.5,34-36
Applications of Solid Lipid Nanoparticles
Oral delivery
Because oral delivery is convenient and well-received by patients, it is a frequently utilized method of drug administration. Solid lipid nanoparticles (SLNs) have the potential to improve medication solubility, stability, and bioavailability, which makes them advantageous for oral drug delivery. SLNs can be taken orally in a variety of forms, such as dispersions, or they can be made into tablets, pellets, capsules, or powders that are packaged in sachets for different dose forms. All things considered, the oral administration of SLNs present a viable strategy for augmenting the oral bioavailability and therapeutic effectiveness of different medications.37,38
Parenteral delivery
Small particles known as SLNs can be injected subcutaneously, intramuscularly, intravenously, or straight into the intended organ. When delivered systemically, SLNs can travel throughout the body, while their removal from circulation is primarily handled by the spleen and liver. When it comes to bodily distribution, SLNs have been found to result in higher drug concentrations in the lung, spleen, and brain. On the other hand, drug dispersion in solution form usually concentrates more on the kidneys and liver. It is important to realize that the information shown here is a general overview and SLN characteristics and applications can vary according on the medicine, formulation, and particular therapeutic goals.5
Topical delivery
Topical delivery is the direct application of substances to the skin for therapeutic or cosmetic reasons. Lipid nanoparticles, more especially, solid lipid nanoparticles, or SLNs have demonstrated potential in topical applications because of their unique characteristics. One of the many benefits of SLNs is its ability to block UV radiation and provide surface protection for the skin. SLNs can be formulated into a range of topical delivery solutions, such as gels, creams, and sprays. SLNs, taken as a whole, offer a promising approach to topical distribution, allowing for the targeted delivery of cosmetic or medicinal substances to the skin's surface with possible benefits such as UV and skin protection.39
SLNs as gene vector carrier
Solid lipid nanoparticles can be used as gene vector carriers in gene therapy. SLNs are intended to introduce genes or nucleic acids (DNA or RNA) into target cells in this particular scenario. To increase the effectiveness of gene transfer, the SLN gene vector can have the diametric HIV-1 HAT peptide T.A.T. 2 added to it. The T.A.T. 2 peptide is derived from the HIV-1 trans activator of transcription (TAT) protein and is widely recognized for its ability to enhance intracellular delivery and cellular uptake of biomolecules. To sum up, SLNs are effective vector carriers for gene therapy. Gene transfer can be aided by the T.A.T. 2 peptide and antibody-lipo polymer conjugates.40
SLNs as cosmeceuticals
In addition to sunscreens and other cosmeceutical products, solid lipid nanoparticles, or SLNs, have been employed as active carriers for molecular sunscreens and UV blockers. Owing to their unique characteristics, these nanoparticles offer several advantages, such as enhanced stability, regulated release, enhanced skin penetration, and focused distribution of active ingredients. All things considered, the addition of SLNs to cosmeceuticals, like sunscreens and creams, may result in better stability, regulated release, and enhanced skin penetration in addition to the delivery of active ingredients to particular body parts. These nanoparticles can help produce cosmetics that are more beneficial to the skin and more effective and efficient.41
SLNs as a targeted carrier for the anticancer drug to solid tumor’s
Solid lipid nanoparticles (SLNs) have shown promise in the targeted delivery of anticancer drugs to solid tumors. As drug carriers, they offer several advantages such as improved drug stability, controlled drug release, and higher drug penetration into tumor tissues. Tamoxifen, a commonly recommended anticancer medication for the treatment of breast cancer, is included in SLNs to guarantee continuous drug release after intravenous administration. Like tamoxifen, SLNs have been employed as carriers for other anticancer drugs such methotrexate and camptothecin to achieve tumor targeting. When methotrexate is loaded into SLNs, it becomes more effective and less harmful to healthy tissues, enabling regulated and targeted delivery. Additionally, camptothecin, a potent anticancer drug, has been encapsulated in SLNs to improve its solubility, stability, and capacity to encourage tumor-specific accumulation. Because they have the ability to prolong drug release, utilize the enhanced permeability and retention (EPR) effect, and enhance medicine stability, they provide promising alternatives for enhancing the effectiveness of cancer treatments while lowering side effects.40-41
SLNs in breast cancer and lymph node metastases
Mitoxantrone-loaded SLN local injections were created to improve the safety and absorption of the drug while reducing toxicity. It has been reported that the effectiveness of SLNs is increased by the addition of doxorubicin (Dox). To make Dox-loaded solid lipid nanoparticles, the Dox was complexed with an anionic polymer derived from soy in the technique and then dispersed with a lipid in water. The efficacy of the system rose and the number of breast cancer cells reduced.41
Stealth nanoparticles
These provide a novel and unique drug delivery method that circumvents the immune system's quick clearance. In theory, these nanoparticles might target certain cells. Research utilizing antibody-labeled stealth lipobodies has shown improved distribution to target tissue's inaccessible areas. Stealth SLNs have been successfully tested with medications and marker molecules in animal models.41 In addition, they can be made more hospitable by the host body by utilizing natural polysaccharides, which will make them more friendly to the body.42-43
Conclusion
Solid lipid nanoparticles (SLNs) provide a feasible therapeutic delivery option for drugs with low aqueous solubility. They can be used in place of conventional colloidal carriers such as emulsions, liposomes, polymeric micro, and nanoparticles. One of the key advantages of SLNs is their ability to encapsulate hydrophobic medications within a lipid matrix, increasing their solubility and stability. This improves these medications' therapeutic efficacy and bioavailability. Furthermore, SLNs provide controlled drug release, allowing for concentrated and prolonged delivery that can enhance therapeutic outcomes and minimize side effects. Furthermore, SLNs may be composed of physiological materials, which qualifies them as biocompatible and suitable for use in human medicine. They are easily produced on a large scale, making industrial processes efficient and successful.
Future Prospects
SLNs possess the capacity to sustain elevated stability throughout their storage duration. Lipids, or oils, and fatty acids come in a wide variety, making it possible to adjust the release kinetics. SLNs are incredibly adaptable lipid carriers that are simple to modify with solid lipid terminal groups to achieve effective enhancement for a particular treatment. Surface modification can effectively handle drug expulsion and targeting issues. SLNs are investigated for their potential as imaging or diagnostic agents in addition to their application in treatment. For the sake of future prospects, the development of surface-modified SLNs should be the exclusive emphasis of front line research. It would be highly beneficial for active and targeted administration in different tissue areas as well as imaging. Young researchers should expect more patented SLN-based (surface-modified SLN) delivery systems shortly for the treatment and detection of various diseases, notably for targeting by modifying the surface. Researchers have already filed and acquired numerous patents related to SLNs. A very well-designed SLN appears to be a potential carrier that could set new standards for medical treatment, diagnosis, and biological medication delivery, if thoroughly investigated.
Conflict of Interest
Nil
Acknowledgements
The authors express their sincere gratitude to the scholars whose works are cited in this research and whose input is greatly appreciated. The authors also acknowledge the publishers, editors, and writers of the books, papers, journals, and other sources that were consulted in order to evaluate and debate the literature for this study.
Supporting File
References
- Nadkar S, Lokhande C. Current trends in novel drug delivery - an OTC perspective. Pharma Times 2010;42 Suppl 4:17-23.
- Loxley A. Solid lipid nanoparticles for the delivery of pharmaceutical actives. Drug Deliv Technol 2009;9 Suppl 8:32.
- Mishra B, Patel BB, Tiwari S. Colloidal nanocarriers: A review on formulation technology, types and applications toward targeted drug delivery. Nanomedicine: NMB 2010;6 Suppl 1:e9-e24.
- Maravajhala V, Papishetty S, Bandlapalli S. Nanotechnology in development of drug delivery system. Int J Pharm Sci Res 2011;3 Suppl 1:84-96
- Ekambaram P, Sathali AH, Priyanka K. Solid lipid nanoparticles: A review. Sci Rev Chem Commun 2012;2 Suppl 1:80-102.
- Muller RH, Mader K, Gohla S. Solid lipid nanoparticles (SLN) for controlled drug delivery - a review of the state of the art. Eur J Pharm Biopharm 2000;50:161-177.
- Mandawgade SD, Patravale VB. Development of SLNs from natural lipids: Application to topical delivery of tretinoin. Int J Pharm 2008;363: 132-138.
- Helgason T, Awad TS, Kristbergsson K, McClements DJ, Weiss J. Effect of surfactant surface coverage on the formation of solid lipid nanoparticles (SLN). J Colloid Interface Sci 2009;334:75-81.
- Sinha VR, Srivastava S, Goel H, Jindal V. Solid lipid nanoparticles (SLN’s) – trends and implications in drug targeting. Int J Adv Pharmaceut Sci 2010;1:212-238.
- Mehnert W, Mader K. Solid lipid nanoparticles-production, characterization and applications. Adv Drug Deliv Rev 2001;47:165-196.
- Muller RH, Radtke M, Wissing SA. Solid lipid nanoparticles (SLN) and nanostructured lipid carriers (NLC) in cosmetic and dermatological preparations. Adv Drug Deliv Rev 2002;54 Suppl 1:131-155.
- Jannin V, Musakhanian J, Marchaud D. Approaches for the development of solid and semi-solid lipid-based formulations. Adv Drug Deliv Rev 2008;60:734-746.
- Manjunath K, Venkateswarlu V. Preparation, characterization, and in vitro release kinetics of clozapine solid lipid nanoparticles. J Control Release 2004;95:627-638.
- Kute VC, Godge G. Preparation & In-Vitro Evaluation of Inclusion Complexes of Simvastatin Tablet with Cyclodextrins. World J Pharm Res 2016;5(2):1022-1041.
- Godge GR, Palwe VD, Pawar PY. Formulation development and in-vitro evaluation of sustained release tablets of telmisartan by solid dispersion technology. Asian J Pharm Technol Innov 2016;04(17):131-139.
- Hiremath S, Godge G, Sonawale B, Shirsath R. Pharmaceutical advances in cyclodextrin inclusion complexes for improved bioavailability of poorly-soluble drugs. Int J Pharm Sci Nanotechnol 2015;8(3):2894-2905.
- Hiremath SN, Kharia AA, Godge GR, Vaidya VR. Studies On The Preparation, Characterisation And Solubility Of Β-Cyclodextrin-Nelfinavir Inclusion Complexes. JPRHC 2010;2(3):279-284.
- Godge G, Labade S. Oral Bioavailability Improvement Using Solid Dispersion Techniques: A Review. Int J Life Sci 2015;1(7):243-252.
- Godge GR, Labade SP. Preparation of Solid Dispersion of Poorly Water Soluble Drug Formulation and Consideration. International J Pharma Sci Res 2015;6(5):897-903.
- Godge GR, Garje MA, Dode AB, Tarkase KN. Nanosuspension Technology for Delivery of Poorly Soluble Drugs and Its Applications: A Review. Int J Pharm Sci Nanotech 2020;13(4):4965-4978.
- Godge GR, Zarekar SB, Padale PJ. Nanosuspension Technologies: An Innovative Approach In Drug Delivery Of Sparingly Soluble Drugs. IJCRT 2021;9(7):409-420.
- Hiremath SN, Kharia AA, Godge GR. Formulation Strategies for Low Absorption Window Antihypertensive Agents. Res J Pharm Techno 2010;03(01):110-118.
- Hiremath S, and Godge G. Preparation and in vitro Evaluation of Inclusion Complexes of Nelfinavir with Chemically Modified β-cyclodextrins. Dhaka Univ J Pharm Sci 2012;11(2):107-116.
- Hu FQ, Yuan H, Zhang HH, Fang M. Preparation of solid lipid nanoparticles with clobetasol propionate by a novel solvent diffusion method in an aqueous system and physicochemical characterization. Int J Pharm 2002;239:121-128.
- Trotta M, Debernardi F, Caputo O. Preparation of solid lipid nanoparticles by a solvent emulsificationdiffusion technique. Int J Pharm 2003;257(1-2): 153-60.
- Yuan H, Huang LF, Du YZ, Ying XY, You J, Hu FQ, et al. Solid lipid nanoparticles prepared by solvent diffusion method in a nanoreactor system. Colloids Surf B Biointerfaces 2008;61:132-137.
- Godge GR, Misal AV, Pawar PY. Formulation and evaluation of mouth dissolving tablet with taste masking resin. Int J Life Sci Rev 2015;1(7): 253-263.
- Sanap DS, Godge GR. Recent trends in medicated chewing gum technology: A review on history, formulation strategies, and characterization. Int J Pharm Sci Rev Res 2019;59(2):72-85.
- Chemate SZ, Godge GR, Pawa KK, Rupnar KA. Preparation and evaluation of hollow calcium pectinate beads for floating-pulsatile drug delivery. Turk J Pharm Sci 2016;13(1):91-102.
- Vaidya VR, Karodi RS, Mohite MT, Hiremath SN, Godge GR. Formulation optimization of mucoadhesive buccal tablets of carvedilol using 32 full factorial designs. Deccan J Pharmaceut Cosmetol 2010;1(2):7-20.
- Shaikh S, Merekar AN, Godge GR, Gaikwad MR. Formulation and in-vitro evaluation of buccal mucoadhesive tablets of captopril by using natural and synthetic polymers. World J Pharm Res 2016;5(7):1296-1315.
- Muller RH, Radtke M, Wissing SA. Solid lipid nanoparticles (SLN) and nanostructured lipid carriers (NLC) in cosmetic and dermatological preparations. Adv Drug Deliv Rev 2002;54 Suppl 1:131-155.
- Jawahar N, Gowthamarajan K, Meyyanathan SN, Soodi S, Lireni CH. Brain delivery by solid lipid nanoparticles for CNS drugs. Int J Pharm Res Dev 2011;3 Suppl 4:206-216.
- Gaumet M, Vargas A, Gurny R, Delie F. Nanoparticles for drug delivery: The need for precision in reporting particle size parameters. Eur J Pharm Biopharm 2008;69:1-9.
- Jores K, Mehnert W, Drechsler M, Bunjes H, Johann C, Mäder K. Investigations on the structure of solid lipid nanoparticles (SLN) and oil-loaded solid lipid nanoparticles by photon correlation spectroscopy, field-flow fractionation and transmission electron microscopy. J Control Release 2004;95:217-227.
- Schubert MA, Muller-Goymann CC. Characterisation of surface-modified solid lipid nanoparticles (SLN): Influence of lecithin and nonionic emulsifier. Eur J Pharm Biopharm 2005;61:77-86.
- Hiremath SN, Godge GR, Kulkarni PS, Landge RB. Discussion on tablets with special emphasis on formulation and coating defects: A review. IJCRT 2021;9(7):124-139.
- Landge RB, Kulkarni PS, Godge GR, et al. A detailed report on tablets with special emphasis on its formulation and manufacturing technology. Int J Creative Res Thoughts 2022;10(3):f624-f641.
- Lippacher A, Muller RH, Mader K. Preparation of semisolid drug carriers for topical application based on solid lipid nanoparticles. Int J Pharm 2001;214: 9-12.
- Godge GR, Hiremath SN. Development and evaluation of colon targeted drug delivery system using natural polysaccharides/polymers. Dhaka Univ J Pharm Sci 2014;13(1):105-113.
- Godge GR, Hiremath SN. Colon targeted drug delivery system: A review. Int J Pharm Drug Ana 2014;2(1):35-48.
- Godge GR, Hiremath SN. An investigation into the characteristics of natural polysaccharide: polymer metoprolol succinate tablets for colonic drug delivery. Mahidol Univ J Pharm Sci 2014;41(2): 7-21.
- Godge G, Hiremath S. Colonic delivery of film-coated meloxicam tablets using natural polysaccharide polymer mixture. Int Curr Pharm J 2012;1(9):264-271