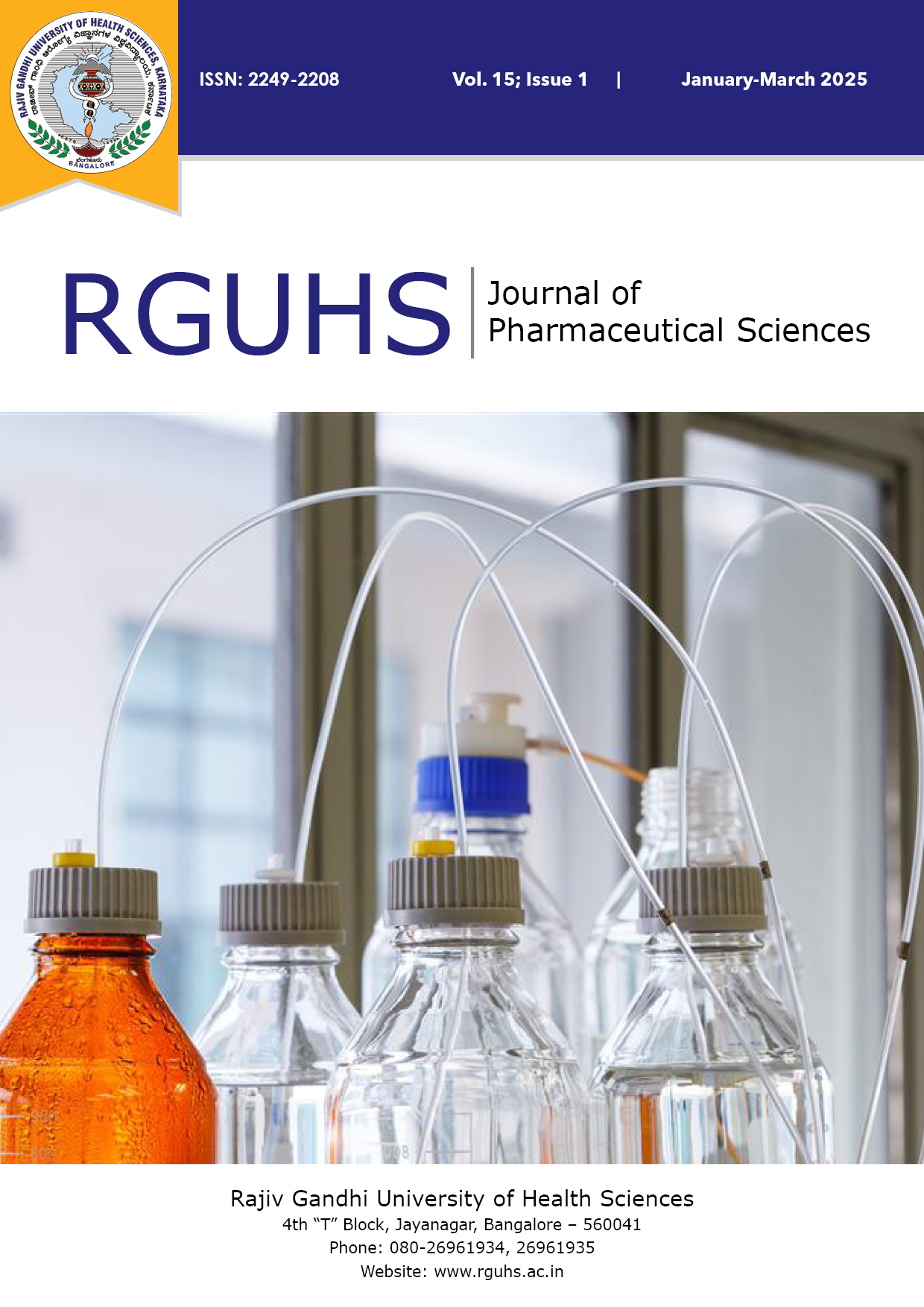
Abbreviation: RJPS Vol No: 15 Issue No: 1 eISSN: pISSN:2249-2208
Dear Authors,
We invite you to watch this comprehensive video guide on the process of submitting your article online. This video will provide you with step-by-step instructions to ensure a smooth and successful submission.
Thank you for your attention and cooperation.
1Padmasree M, Department of Pharmaceutics, Aditya Bangalore Institute of Pharmacy Education & Research, Yelahanka, Bangalore, 560064, India.
2Department of Pharmaceutics, Aditya Bangalore Institute of Pharmacy Education & Research, Yelahanka, Bangalore, 560064, India.
*Corresponding Author:
Padmasree M, Department of Pharmaceutics, Aditya Bangalore Institute of Pharmacy Education & Research, Yelahanka, Bangalore, 560064, India., Email: padmasree.mpharm@gmail.com
Abstract
Background: Liposomes are lipid aggregates with a careful choice of lipid composition and process parameters. Liposomes are formulated by optimization of process parameters of liposomes to achieve desired bioavailability and to improve stability.
Objectives: The main objective of the present research was to formulate colon targeting capecitabine loaded stealth liposomes, characterized by using Nuclear magnetic resonance (NMR) techniques to determine the fractions of the drug, capecitabine (CAP), encapsulated in the liposomal suspension.
Methods: Liposome properties were evaluated by NMR. 31P-NMR (31Phosphorus NMR) in combination with the use of chemical shift reagents were used for the identification of lamellarity of liposomes which can have a strong effect on both encapsulation efficiency and the efflux rate of encapsulated active compounds.
Results: The results suggested that capecitabine remained intact during the loading process, and the liposomal drug was present in a soluble form. Conclusion: 31P-NMR was found to be an effective method to study the lamellarity of liposomes and to determine the distributed fractions of encapsulated capecitabine.
Keywords
Downloads
-
1FullTextPDF
Article
Introduction
A liposome is a spherical shaped vesicle with a membrane made up of a phospholipid and cholesterol bilayer used for the delivery of drug.1,2 Liposomes are mostly used for drug delivery and biosensing applications.3−5 Liposomes form spontaneously when particular lipids are hydrated in aqueous media. Liposomes are ideal candidates for biological applications due to their intrinsic biocompatibility and chemical versatility.6,7 Liposomes can accommodate both water and lipid soluble drugs.8,9 While designing liposomes several parameters have to be considered and they can be differentiated based on the method of preparation and their physical characteristics.10 In all the different physical characteristics of liposomes, vesicle lamellarity is very important as it affects the efflux rate and encapsulation efficiency of the liposomal encapsulated drugs which have the implications on drug delivery and stability of the liposomes.11,12
Liposomes can be classified based on their structural features as, small unilamellar vesicles (SUVs) with the diameter in the range of 20-40 nm, large unilamellar vesicles (LUVs) with the diameter larger than 100 nm, and the multilamellar vesicles (MLVs) whose size can be ranging from 0.1 to several μm.13 SUVs can be characterized both by the high curvature and by the marked packing asymmetry of the inner and outer monolayers.14-16 Because of these structural properties, SUVs are significantly different from the biological cell membranes. LUVs constitute a good model for the biological membranes due to their smaller curvature and also are good candidates for therapeutic delivery applications.17 MLVs have very low curvature, extremely near to that of the real biological membranes, and therefore are useful for membrane characterizations.18,19 The most dynamic tool for evaluation of liposomal formulations is Nuclear magnetic resonance (NMR).20,21 NMR applications are more beneficial because this method is based on the use of intrinsic reporter moieties (the various nuclei, i.e., 13C, 31P, 1H, 15N, 195Pt). In case of capecitabine-loaded liposomes, NMR provides important information on the liposomal phospholipids.22-24 By using NMR study, we characterized the physical and chemical nature of capecitabine in the liposomal formulations.
NMR resolution differs among liposomal vesicles because of their different characteristic structures. SUVs, show fast molecular tumbling averages, and thus allow classical NMR spectra with good resolution.25,26-28 Molecular tumbling is slow in LUVs and MLVs to average out anisotropic interactions and therefore the NMR spectra appears to be broad and unresolved.
Advanced techniques are required to determine the degree of multilamellarity. Among these, Phosphorus NMR (31P NMR) has been used to estimate vesicle lamellarity. Different methods are available in the liposome formulation and each of these methods produce a different system with respect to vesicle lamellarity and size distribution. The thin film hydration method described for liposome preparation involves formation of dried lipid film in a round-bottom flask, which is then allowed for hydration with phosphate buffer, followed by sonication.29,30,31 This method yields multilamellar vesicles in the micron-size range with a heterogeneous size distribution. Size reduction techniques are used to obtain homogeneous small unilamellar vesicle populations such as extrusion through a membrane by using pore size below 200 nm or sonication. Particle size of liposomes, hydration level have significant effect on the NMR spectra.32
Materials and Methods
For the preparation of liposomal formulation, capecitabine was received as a gift sample from Mylon Laboratories, Bangalore, India. DSPC (Distearoylphosphatidyl choline) and Polyethylene glycol–phosphatidylethanolamine (PE- PEG) 2000 were purchased from Lipoid Germany. Solvents and reagents used were of laboratory reagent (LR) grade. All other reagents and chemicals used were of the analytical grade.
Preparation of capecitabine encapsulated stealth liposomes
PEGylated liposomes of capecitabine were prepared by thin film hydration method from various combinations of phospholipids. The weighed quantity of drug, lipids and cholesterol was dissolved in organic solvents like ethyl acetate and ethanol (2:1) in a round bottom flask. Round bottom flask was attached to a rotary evaporator by means of a clip, and vacuum was applied through a vacuum pump, rotated at 80 rpm and subjected to evaporation to obtain a thin dry lipid film. Lipid film was completely dried overnight to drain residual organic solvent by using vacuum pump. The film was allowed to hydrate using Phosphate-buffered saline (PBS) pH 7.4 above transition temperature by hand shaking for 15 minutes and further kept at room temperature and subjected to sonication. The non-entrapped drug was separated by centrifugation. Final liposomal dispersion was packed in sterile glass vials and covered with special stoppers for lyophilization. Liposomal dispersion was preserved by addition of sodium azide.33
Nuclear Magnetic Resonance
Solid State NMR Analysis
Capecitabine-loaded liposomes were studied by 31P NMR. Capecitabine loaded liposomal formulations were formulated and analyzed using solid-state 31P NMR. The analyses were performed at a spinning speed of 10 KHz. 31P measurements were carried out at room temperature using a 5 mm computer-switchable probe. The 31P chemical shifts were measured relative to phosphoric acid set at 0 ppm. Data were collected with broad-band decoupling of the protons, with spectral width of 10 KHz, and an acquisition time of 1.6 s. The liposomes were composed of DSPC (Distearoylphosphatidyl choline), PE 18:0/18:0 PEG 2000 and cholesterol. Samples was prepared by mixing 700 Wl of the original liposome sample with 70 Wl of 2H2O. The 31P-NMR is the most accurate method for determining the lamellarity of liposomes; the broad peak spectrum designates the presence of MLV while a narrow peak spectrum indicates SUV. Using paramagnetic ions such as Mn2+, Co2+, and Pr3+ shifts the individual peaks to either downfield or upfield because of ionic interactions towards the phosphate backbone.34
Results
Solid State NMR Studies
To analyze the localization of the capecitabine in the liposomal formulations, 31P solid state NMR studies were carried out. Solid state 31P NMR studies provide valuable information regarding the structure and dynamics of the lipid membrane and the phase in which the lipid molecules are present in, and any possible interaction between the drug molecule and the membrane. Figure 1 shows the solid state 31P NMR spectra for the capecitabine-loaded stealth liposomes. Phospholipid structure DSPC constituent used for the formation of liposomes is represented in Figure 1(a). Apart from the DSPC, PE-PEG and cholesterol were observed in Figure 1 (b & c). The spectra for the capecitabine loaded liposome states that capecitabine does not interact with the hydrophobic fatty acid region of the liposome bilayer. Considering Figure 1, signals observed at 1.00, 0.980, 7.103 and 2.027, 0.994, 0.612, 1.336, 2.027, 8.166, 0.157, 1.00, 7.013 ppm are corresponding to R -NH2, CH3, Ar-OH, R-NH2, R-OCH3, RCH3, Ar-phenyl oxo, Phosphate (PO42) RCH3, R-CH2, HC-OH, respectively. In the capecitabine-loaded liposomes, broadening of the peak suggests that capecitabine localizes near the phosphate moiety. At room temperature, the 31P peak observed was very broad.
Discussion
Liposomal formulations must be stable and should have a long circulation time to improve therapeutic action. In the present research work, we used 31P-NMR to determine the concentration of encapsulated capecitabine liposomes, and any other phosphorus containing drugs in the aqueous liposomal core. Vesicle size of the liposomes should be small enough to extravasate into the tissue. Through the application of NMR techniques, 31P NMR, capecitabine-containing liposome preparations were observed to have identical lipid concentrations and similar size distribution. This suggests that no possible interactions occurred between the capecitabine and the phospholipid. The use of 31P-NMR in combination with chemical shifting reagents has been extensively described. Encapsulated capecitabine has a characteristic peak that was slightly shifted downfield. This shift was caused by the physicochemical environment induced by the interior lipid bilayer membrane, resulting in de-shielding of the phosphorus nuclei shifting its resonance downfield.
Conclusion
It can be concluded that NMR is a powerful tool in the characterization of drug-lipid interactions and for the characterization of physical state of capecitabine inside the liposomes. Even after the drug encapsulation, capecitabine remained unaffected during the loading process. Encapsulated capecitabine resembles, with minor differences, the parent compound dissolved in the aqueous phase. That is, the drug within the liposomes did not precipitate or crystallize. Drug encapsulated in the liposomes was very stable with no efflux of drug or influx of medium components observed. The formulation was stable and no leakage of the hydrophilic capecitabine was detected under the experimental conditions. Improved capecitabine solubility by the contribution of the membrane grafted PE-PEG 2000 and possibly by the small size of the internal aqueous compartment was observed.
Conflict of Interest
None
Acknowledgments
I would like to Heart fully thank my guide Dr. B A Vishwanath for his continuous, enormous support and encouragement.
Supporting File
References
- Bangham AD, Horne RW. Negative staining of phospholipids and their structural modification by surface-active agents as observed in the electron microscope. J Mol Biol 1964;8: 660-668.
- Papahadjopoulos D. (ed.) Liposomes and their uses in biology and medicine. Ann NY Acad Sci 1978;308:1-462.
- Mazur F, Bally M, Stadler B, Chandrawati R. Liposomes and lipid bilayers in biosensors. Adv Colloid Interface Sci 2017;249:88–99.
- Pattni BS, Chupin VV, Torchilin VP. New developments in liposomal drug delivery. Chem Rev 2015;115:10938–10966.
- Liu Q, Boyd BJ. Liposomes in biosensors. Analyst 2013;138:391–409.
- Wasan KM, Lopez-Berestein G. The past, present, and future uses of liposomes in treating infectious diseases. Immunopharm Immunotox 1995;17:1-15.
- Gregoriadis G. Engineering liposomes for drug delivery: progress and problems. TIBTECH 1995;13:527-537.
- Mura S, Nicolas J, Couvreur P. Stimuli-responsive nanocarriers for drug delivery. Nat Mater 2013;12(11):991-1003.
- Zucker D, Marcus D, Barenholz Y, Goldblum A. Liposome drugs’ loading efficiency: A working model based on loading conditions and drug’s physicochemical properties. J Controlled Release 2009;139:73–80.
- Fatouros DG, Antimisiaris SG. Effect of amphiphilic drugs on the stability and zeta-potential of their liposome formulations: a study with prednisolone, diazepam, and griseofulvin. J Colloid Interface Sci 2002;251:271–277.
- Frohlich M, Brecht V, Peschka-Süss R. Parameters influencing the determination of liposome lamellarity by 31P-NMR. Chem Phys Lipids 2001;109:103–112.
- Duplessis J, Ramachandran C, Weiner N, Muller D. The influence of lipid composition and lamellarity of liposomes on the physical stability of liposomes upon storage. Int J Pharm 1996;127: 273–278.
- Lasic DD. The mechanism of vesicle formation. Biochem J 1988;256:1–11.
- Schuh JR, Banerjee U, Müller L, Chan S. The phospholipid packing arrangement in small bilayer vesicles as revealed by proton magnetic resonance studies at 500 MHz. BBA– Biomembranes 1982;687:219–225.
- Longmuir KJ, Dahlquist FW. Direct spectroscopic observation of inner and outer hydrocarbon chains of lipid bilayer vesicles. Proc Natl Acad Sci USA 1976;73:2716–2719.
- Tauskela JS, Thompson M. A 31P-NMR spin-lattice relaxation and 31P{1H} nuclear Overhauser effect study of sonicated small unilamellar phosphatidylcholine vesicles. BBA–Biomembranes 1992;1104:137–146.
- Vemuri S, Rhodes CT. Preparation and characterization of liposomes as therapeutic delivery systems: a review. Pharmaceut Acta Helv 1995;70:95–111.
- Smith RL, Oldfield E. Dynamic structure of membranes by deuterium NMR. Science 1984;225:280–288.
- Forbes J, Bowers J, Shan X, Moran L, Oldfield E, Moscarello MA. Some new developments in solid state nuclear magnetic resonance spectroscopic studies of lipids and biological membranes, including the effects of cholesterol in model and natural systems. J Chem Soc Faraday Trans 1988;84:3821–3850.
- Dong RY. Nuclear magnetic resonance of liquid crystals. New York: Springer-Verlag; 1997.
- Evans FD, Wennerstrom H. The colloidal domain, where physics, chemistry, biology and technology meet. New York: VCH Publishers; 1994.
- Batool F, Emwas AH, Gao X, Munawar MA, Chotana GA. Synthesis and suzuki cross-coupling reactions of 2,6-bis(trifluoromethyl) pyridine-4-boronic acid pinacol ester. Synth Stuttg 2017;49:1327–1334.
- Elbaz AM, Gani A, Hourani N, Emwas AH, Sarathy SM, Roberts WL. TG/DTG, FT-ICR mass spectrometry, and NMR spectroscopy study of heavy fuel oil. Energy Fuels 2015;29:7825–7835.
- Ameel AGA, Van Oudenhoven V, Emwas AH, Sarathy SM. Predicting octane number using nuclear magnetic resonance spectroscopy and artificial neural networks. Energy Fuels 2018;32:6309–6329.
- Rehman ZU, Jeong S, Tabatabai SAA, Emwas AH, Leiknes T. Advanced characterization of dissolved organic matter released by bloom-forming marine algae. Desalin Water Treat 2017; 69:1–11.
- Ye T, Mo HP, Shanaiah N, Gowda GAN, Zhang SC, Raftery D. Chemoselective N-15 Tag for sensitive and high-resolution nuclear magnetic resonance profiling of the carboxyl-containing metabolome. Anal Chem 2009;81:4882–4888.
- Kaplan O, van Zijl P, Cohen JS. Information from combined 1 H and 31P NMR studies of cell extracts: Differences in metabolism between drug-sensitive and drug-resistant MCF-7 human breast cancer cells. Biochem Biophys Res Commun 1990;169:383– 390.
- Chapman D, Kamat VB, De Gier J, Penkett SA. Nuclear magnetic resonance studies of erythrocyte membranes. J Mol Biol 1968;31:101–114.
- Bhamidipati SP, Hamilton JA. Interactions of lyso 1-palmitoylphosphatidylcholine with phospholipids: A 13C and 31P NMR study. Biochemistry 1995;34:5666–5677.
- Traikia M, Langlais BD, Cannarozzi G, Devaux PF. High-resolution spectra of liposomes using MAS NMR. The case of intermediate-size vesicles. J Magn Reson 1997;125:140–144.
- Oldfield E, Bowers JL, Forbes J. High-resolution proton and carbon-13 NMR of membranes: why sonicate? Biochemistry 1987;26:6919–6923.
- Bloom M, Burnell EE, MacKay A, Nichol CP, Valic MI, Weeks G. Fatty acyl chain order in lecithin model membranes determined from proton magnetic resonance. Biochemistry 1978;17:5750–5762.
- Celik B, Sagıroglu AA, Ozdemir S. Design, optimization and characterization of coenzyme Q10- and D-panthenyl triacetate-loaded liposomes. Int J Nanomedicine 2017;12:4869–4878.
- Peleg-Shulman T, Gibson D, Cohen R, Abra R, Barenholz Y. Characterization of sterically stabilized cisplatin liposomes by nuclear magnetic resonance. Biochim Biophysic Acta 2001;1510:278-291