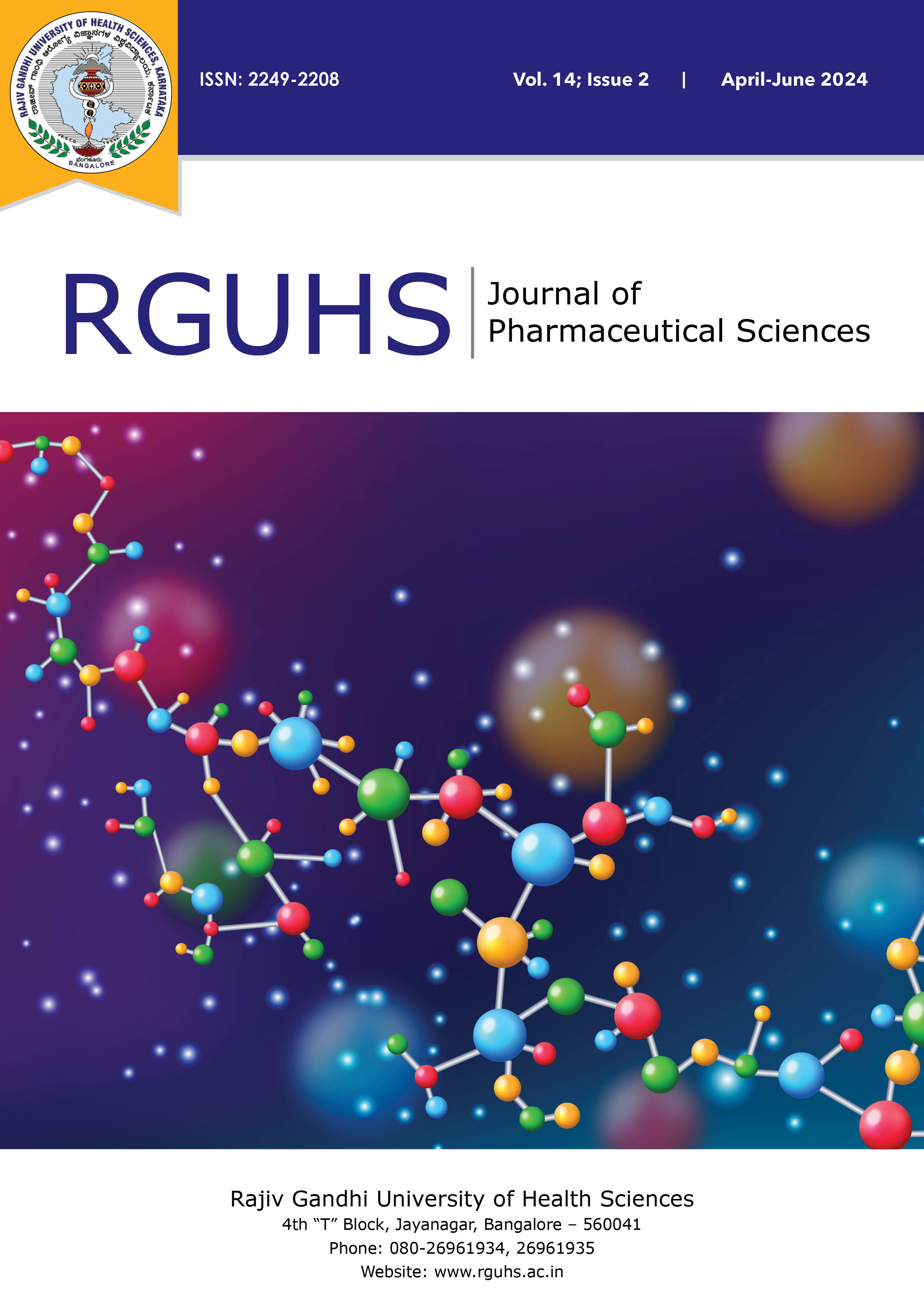
RJPS Vol No: 14 Issue No: 2 eISSN: pISSN:2249-2208
Dear Authors,
We invite you to watch this comprehensive video guide on the process of submitting your article online. This video will provide you with step-by-step instructions to ensure a smooth and successful submission.
Thank you for your attention and cooperation.
Manjunatha Kattalagere Maheswarappa*, Juturu Thimmasetty, Ranjeet Singh, Shashank Nayak Nagar
Bapuji Pharmacy College, Davangere & Rajiv Gandhi University of Health Sciences, Bangalore, Karnataka
Author for correspondence
Manjunatha Kattalagere Maheswarappa
Associate Professor
Dept. of Pharmaceutics
Bapuji Pharmacy College
S.S. Layout, A-Block, Shamanur Road, Davangere – 577004
Mail I.D: katmanju@gmail.com

Abstract
An attempt is made to determine the solubility parameter of moxifloxacin HCl and to establish the correlation between solubility parameter and drug properties using different methods given in the literature. The solubility parameter of the drug was calculated by Hoy’s molar attraction constants and peak solubility technique. Saturation solubility values of the drug were determined in various solvent blends having solubility parameter values ranging from 8.9 to 20.7 H. The solubility parameter of drug was 11.46 H and 11.67 H from Hoy’s method and peak solubility method, respectively. Minimum inhibitory concentration (MIC) and Zone of inhibition (ZOI) of the drug for E. coli, S. aureus, and S. Paratyphi-A were determined. MIC was maximum for the drug in solvent system having the solubility parameter nearer to that of the drug. Minimum ZOI was observed for the drug in solvent system having the solubility parameter nearer to the drug solubility parameter. Transport studies revealed that the drug rejection from the solvent blends was dependent on its solubility parameter. Correlation was observed between the solubility parameter of the drug and the properties namely solubility, diffusion of the drug, MIC, and antibacterial activity. Thus the solubility parameter of the drug can be correlated with the antibacterial activity of drug, which can be used as a model.
Keywords
Downloads
-
1FullTextPDF
Article
INTRODUCTION
The solubility parameter (δ) is an intrinsic physicochemical property of a substance that expresses the cohesive forces between like molecules.1 It is defined as the square root of the cohesive energy density of the substance. It expresses the cohesive forces between like molecules and the cohesion energy is partitioned in terms of nonpolar or dispersion interaction, Ed , permanent dipole-dipole (polar) interaction, E p , and hydrogen bonding, Eh .
Solubility parameter has several applications in pharmaceutical industry. The solubility parameter has been used to explain drug action2 , structure– activity relationships3 , drug transport kinetics4 , and in situ release of drugs.5
A good correlation was observed between the solubility parameter of barbiturates and their reported pharmacological activity. In gambusia fish, log linear relationships were found between the absorption parameters of some barbiturates and their solubility parameters.6 It is a valuable tool for understanding plant surface interaction.7 Solubility parameter governs the self-assembly of molecular gels using 1,3:2,4-dibenzylidene sorbitol as the gelator.8 Role of solubility parameter for predicting the solubility of a drug in solvent/ solvent blends was reported.9 Influence of partial solubility parameters on the solubility behaviour of pimozide was explained.10 The knowledge of three-dimensional Hansen solubility parameters as predictors of miscibility of coformer and drug is helpful in the cocrystal formation.11
To explain structure activity relationship, solubility parameter was used as a substitute for partition coefficient. The mechanism of narcosis was explained using the solubility parameter as narcotic action of certain drugs depends on cohesive energy density. Interaction of solute and solvent influence the dissolution of drug molecules, leads to rejection of solute molecules in favour of absorption or membrane penetration. All these applications reveal the need for evaluating solubility parameter values for drug substances, solvents/vehicles, and biological membranes. These parameters are helpful in better designing of suitable dosage forms. Several methods are explained in the literature to determine the solubility parameter of the drugs.12
Moxifloxacin HCl, a model molecule of the present investigation, is a fourth generation fluoroquinolone with expanded activity against gram-positive bacteria as well as atypical pathogens. The drug binds and inhibits the bacterial enzymes DNA gyrase (topoisomerase II) and topoisomerase IV, resulting in inhibition of DNA replication and repair cell death in sensitive bacterial species.13
The present work describes the new application of the solubility parameter for predicting the antibacterial activity of moxifloxacin HCl prepared in the solvents of different polarity. Physical models like solubility and transport studies and biological models like minimum inhibitory concentration (MIC) and zone of inhibition were used to establish correlations. This biophysical modelling is a novel model, as the regulation restricts the use of animals for research work.
MATERIALS AND METHODS
Materials:
The moxifloxacin HCl was obtained as a gift sample from Micro Labs, Bangalore, India and was authenticated using FTIR and DSC. Mac Conkey Agar medium, Mannitol salt agar medium, Xylose lysine deoxycholate medium, nutrient broth, and dialysis membrane were purchased from HiMedia Labs, Mumbai, India. Staphylococcus aureus (NCTC No.6571), Salmonella paratyphi-A (ATCC No. 9150) and Escherichia coli (ATCC No.10536) were obtained from Mumbai, India. Double distilled water was used for experimental purpose throughout the study. Other chemicals were of analytical grade and used as received.
Theoretical determination of the solubility parameter:
Literature survey revealed that solubility parameter of moxifloxacin is not estimated by any method till date. In this method, the ring structure of moxifloxacin was opened to produce an open chain structure for convenience, as shown in Fig.1. Then, appropriate constants were assigned to substituents.14 The solubility parameter of moxifloxacin (δ) was calculated using Eq.
δ = ∑∆ U/ ∆∑ V (1)
where in ∑∆∆U is the sum of energy constants of the substituents and∑∆V is the sum of the molar volumes of the substituents.
Solubility parameter using theoretical molar volume is 8.78 H (18 MPa1/2) and using experimental molar volume is 11.46 H (23.48 MPa1/2).
Estimation of moxifloxacin HCl:
The moxifloxacin HCl stock solution (100 µg/ml) was prepared in 0.1N HCl. Different aliquots were prepared from stock solution (2, 4, 6, 8, 10, and 12 µg/ml). Absorbances were measured at 295.60 nm using an UV spectrophotometer (Shimadzu 1700, Kyoto, Japan). The Beer-Lambert law obeyed in this concentration range (R²=0.9998). The regression equation, y = 0.0976x+0.0036, was used for estimating the concentration of moxifloxacin HCl in the entire research work. Calibration curve for moxifloxacin HCl, constructed in a similar manner, in phosphate buffer solution (pH 7.4) at an analytical wavelength of 288.60 nm was also used for estimating the drug content as per the need arises. The regression equation is y = 0.0883x+0.0075and R² = 0.9995.
Solubility:
The solubility of moxifloxacin was determined in solvent blends of ethyl acetate-propylene glycol (100-00, 75-25, 50-50, 25-75% ethylacetate-propylene glycol) and propylene glycol-water (100- 00, 75-25, 50-50, 25-75% propylene glycol-water). This combination of solvent systems exhibits extremities of polarity. The solubility parameter of the solvent blends ranges from 8.9 to 20.7 H. Around 10 ml of the solvent blend was taken in a set of volumetric flasks to which sufficiently excess quantity of moxifloxacin was added and the flasks were subjected to shaking at constant speed of 150 rpm in a shaker bath maintained at a temperature of 25±1 °C for 72 hours. Preliminary studies showed that this time was sufficient to ensure saturation equilibrium.15,16 After equilibrium was established, solutions were filtered through Whatman filter paper and the filtrate was suitably diluted with the solvent used for the calibration curve and the concentration of moxifloxacin HCl was determined spectrophotometrically. All experimental results were expressed as the average of at least three determinations. The mole fraction solubility of moxifloxacin HCl was calculated using the number of moles of moxifloxacin HCl and solvents in the mixtures.17,18,19
Transport studies:
The kinetic model was used for transport studies. The apparatus consists of donor and receptor compartments. Donor compartment was a 19.4 cm long borosilicate glass tube of 2.3 cm internal diameter. Receptor compartment was a 100 ml borosilicate glass beaker. One end of the donor compartment was covered with a dialysis membrane, which was previously hydrated overnight. Five ml of the solution containing 100 µg/ml of the drug was placed in the donor compartment and was immersed partially in the receptor compartment containing 50 ml phosphate buffer solution pH 7.4. The solution in the receptor compartment was stirred at a constant rpm using a magnetic stirrer maintaining the constant temperature of 37±1 °C. The samples of 5 ml solution were withdrawn from the beaker at different intervals up to 6 hours during the transport process. Each time, fresh phosphate buffer solution (pH 7.4) was replaced. These transport studies were conducted in triplicate. The drug concentration was analysed spectrophotometrically. The experiments were repeated in a similar manner using the different solvent mixtures (solubility parameter range of 8.9 to 20.7 H) containing the drug solution.20
MIC of moxifloxacin HCl:
The MIC values of moxifloxacin HCl in the different solvent blends were determined for one gram positive bacterium and two gram negative bacteria (Staphylococcus aureus, Salmonella paratyphi-A, and Escherichia coli) by the tube dilution method. Mac Conkey agar medium for E.coli, mannitol salt agar medium for S.aureus, and xylose lysine deoxycholatemedium for S.paratyphi-A were used. A sterile stock solution of moxifloxacin HCl (160 µg/ml) was prepared in the solvent blends. To 2 ml of each medium, moxifloxacin HCl solution was added to give concentration of 80 µg/ ml. Additional different concentration solutions (40,20,10, 5, 2.5, 1.25, 0.625, 0.312, and 0.156 µg/ml) were prepared by serial dilution with the medium. These tubes were then inoculated with standard inoculums of the respective test organisms, with the inoculum level being the same in all the tubes. Positive controls (medium inoculated with culture, solvent mixture excluding drug added), and negative controls (medium without culture and drug solution) were also incubated simultaneously. Triplicate studies were conducted for each organism under study in different solvent mixtures. MIC values for moxifloxacin HCl in eight solvent blends were measured.21
Zone of inhibition of moxifloxacin HCl:
The antibacterial activity of moxifloxacin HCl was studied by the diffusion method using the specific media as mentioned earlier for the three microorganisms. Moxifloxacin HCl solutions (300 µg/ml) were prepared in eight solvent blends. Medium was prepared and 100 ml aliquots of each were distributed into 250 ml conical flasks. The media were sterilized by autoclaving. Media were inoculated with one ml of standard inoculums and transferred to petri dishes. After solidification, cups were made in the agar with a sterilized cork borer. The cups were well spaced to avoid overlapping of inhibitions. The cups were filled with 0.1 ml of moxifloxacin HCl stock solution and incubated for 24 hours. Zones of inhibition were measured using the Hi Antibiotic Zone Scale (Himedia).Triplicate studies were conducted for each organism under study in the solvent blends. Positive and negative controls similar to those mentioned above were also incubated simultaneously.21
RESULTS AND DISCUSSION
Solvent mixtures of EA-PG and PG-water were used in different concentrations. The solubility parameter exhibited by these solvent blends range from 8.9 to 20.7 H. Majority of the drugs fall within this range.
Molar volume:
From the solvent displacement method it was found that equivalent volume of n-hexane displaced by 500 mg of drug is 0.3263 ml. Thus, the density of moxifloxacin HCl is 1.5262 g/ml, and the molar volume of moxifloxacin HCl is therefore [(MW) 437.89/1.5262] = 286.9191 ml/mol. From the average of the three trials, the molar volume of moxifloxacin HCl was obtained as 287.3249 ml/ mol.
Solubility parameter:
As shown in Table 1, and according to Hoy’s method, based on experimental molar volume (287.32 ml/mol) of moxifloxacin HCl, the solubility parameter of moxifloxacin HCl was predicted as 11.46 H. As shown in Table 2, eight different solvent blends were prepared using the three solvents EA, PG, and water. The solubility parameters of solvent mixtures (δ1 ) were calculated from the following Eq. 2.
δ_1=[δ(“solvent A”)×φ(“solvent A”)]+[δ (“solvent B”)×φ(“solvent B”)]”/ “φ(“solvent A”)+φ(“solvent B”) in which δ1 is the solubility parameter of the solvent mixtures, and δ and φ denote the solubility parameter and volume fraction of the corresponding pure solvents, respectively.The experimental mole fraction solubility of moxifloxacin HCl in various solvent mixtures is reported in Table 2.
Experimental determination of solubility parameter was based upon the maximum solubility of moxifloxacin in solvent blends. A plot of the mole fraction solubility of moxifloxacin HCl against the solubility parameter of solvent mixtures is shown in Fig.2. Inverse parabolic response was observed. Evaluation of solubility parameter in different solvent blends of various polarities would provide important insight about the solubility of drug. As the solubility parameter of solvent mixtures increases from 8.9 H, the mole fraction solubility of moxifloxacin HCl increases, and this trend continued until the solubility parameter reaches 11.67 H. Thereafter, further increase in the solubility parameter of solvent mixtures, there was a decrease in mole fraction solubility of moxifloxacin HCl. The peak solubility was observed at 11.67 H. Therefore the δ2 value of moxifloxacin HCl was identified as 11.67 H. The maximum mole fraction solubility of moxifloxacin HCl at the peak of the curve shown in Fig. 4 indicates that there may be selfassociation of moxifloxacin HCl or of the solvent, or both. Emami et al22 reviewed the mechanism of bactericidal activity of many quinolones and reported that it occurs by self-association of two molecules and simultaneous inhibition of DNA gyrase or DNA type IV topoisomerase.
Transport studies of moxifloxacin HCl:
The transport profiles of moxifloxacin HCl in two sets of solvent mixtures are shown in Fig.3 (ethyl acetate-propylene glycol) and Fig.4 (propylene glycol-water). For better interpretation, a plot is drawn taking solubility parameter of the solvent blends on x-axis and mole fraction solubility/cumulative transport of moxifloxacin HCl (six hour data) on y-axis as shown in the Fig.5. Perusal to the Fig.5, it was observed that a gradual decrease of drug transport from the solvent blends of solubility parameter up to 11.67 H (trough) whereas drug transport gradually increased thereafter upon increase in the solubility parameter values of solvent blends. This indicates aparabolic profile of transport with atroughat δ1 =11.67 H. This trough value was nearer to the δ2 value of moxifloxacin HCl (Hoy’s method using experimental molar volume), i.e., 11.46H. This empirical observation indicated that the higher the miscibility of drug and solvent system, lower the drug transport.
According to regular solution theory, if |δ2 - δ1 |=0, then the interactions between solute and solvent are stronger.7 In other words, when |δ2 -δ1 |=0, the mutual solubility increases. This phenomenon is required for the dissolution of drugs. When the solubility of a drug is high, the solvent does not reject the drug molecules and hence, the release of drug decreases. The term |δ2 -δ1 | represents the delivery vehicle rejection factor. The higher the difference |δ2 -δ1 |, the better is the rejection of drug molecules by the solvent (vehicle). These rejected drug molecules can easily be transported through bio-membranes. Fig. 5 reveals that the maximum solubility of the drug in the solvent blend was observed at 11.67H, which was the point where minimum transport of the drug was observed. This observation matches with the fundamental principle of solubility.
MIC of moxifloxacin HCl:
The role of solvent blends on the minimum inhibitory concentration (MIC) of the drug was investigated. Positive controls showed that the solvent mixtures used did not have any effect on bacterial growth. The MIC of drug was 300 µg/ml under the experimental conditions. This MIC value was used for selecting a suitable concentration of drug for antibacterial studies. Instead of MIC, the reciprocal of MIC was considered for correlation. The general principles suggested that the higher the value of 1/MIC, greater is the activity and the higher is drug rejection by the solvent system. The MIC and 1/MIC results for the drug with E.coli, S.aureus, and S. paratyphi-A for different solvent mixtures, are reported in Table 3.
Perusal to the Fig. 6 indicates that parabolic response was observed with the S.aureus microorganisms i.e., higher the 1/MIC value, greater the activity was observed. In specific, EA-PG and PG-Water systems provided graded responses for all the microorganisms. The inflection observed at 11.67 H is matching with the solubility parameter of drug determined by methods explained earlier. Further, vehicle rejection factor |δ2 -δ1 | was verified with (1/MIC) values. The graph of % CDT and 1/MIC versus solubility parameter of solvent blends for S.aureus is shown as a sample representation in the Fig. 6. Similar observations were made with other two microorganisms.
Solubility and zone of inhibition of moxifloxacin HCl:
The influence of the solvent mixture on the antibacterial activity of drug was evaluated using the agar diffusion method. The drug concentration was maintained constant, so that the possible influence of a concentration gradient was ruled out. Hence, solvent-solute interactions become a factor in the observed antibacterial activity. Since moxifloxacin HCl interacts with intracellular system (DNA gyrase), the zone of inhibition should reflect the solubility parameter requirement for the DNA gyrase drug interaction. But, several processes, including rejection by the delivery vehicle (solvent), transport (by diffusion), and permeability across the cell membranes are prerequisites, for the drug to react with the enzyme system; the present studies provide prima facei evidence.
The data of zone of inhibition obtained for three organisms are tabulated in Table 4. The profile was recorded against the solubility parameters of vehicles in Fig. 7. In all the three organisms, a parabolic relationship was observed with a trough at 11.67 H. The zones of inhibition values were graded reflecting the usefulness of the data for the correlation.
In spite of these difficulties, the rejection factor of vehicle was considered for analysis. Data were reported in Table 4 and a plot was recorded in Fig. 8. The zone of inhibition was very high when |δ2 -δ1 |> 0.21 H. The activity responses are similar to one another. Beyond |δ2 -δ1 | value of 0.21 H units, as the difference increases, the zone of inhibition increases, due to enhanced rejection of drug by vehicles. Thus, zone of inhibition would be a better model for highlighting the vehicle rejection property.
The solubility data and zone of inhibition of drug from different solvent mixtures, in the case of S.aureus, are plotted against solubility parameter in Fig. 9. As the solubility parameter of the solvent mixtures increases from 8.9 H, the zone of inhibition of drug decreases and a minimum zone of inhibition is observed at the trough in the curve (Fig.9) at 11.67 H. Further increase in the solubility parameter for the solvent mixture corresponds to increase in the zone of inhibition. The zones of inhibition values were graded, reflecting the usefulness of the data used for the correlation. A perusal of Fig. 9 indicated that the higher the solubility, the lower is the zone of inhibition. A high solubility implies strong drug-solvent interactions. This results in a decreased availability of the drug for diffusion and permeability through the cell wall. This may be due to decreased transport as indicated in Fig. 10. Cumulative transport after 6 hours and the zone of inhibition of moxifloxacin HCl for different solvent mixtures, in the case with S.aureus are plotted against the solubility parameter as shown in Fig. 10. The trend clearly demonstrated that, as the transport of moxifloxacin HCl decreased, the zone of inhibition also decreased. This is because the rate of drug transport (diffusion) influences the membrane permeability and subsequently the enzyme interaction. Similar trends were also observed for E.coli and S.paratyphi A.
Moxifloxacin HCl shows antibacterial activity by suppressing DNA gyrase (main bacterial enzyme) that frigid the chromosomal DNA and leads to cell death. According to this situation, the solvent blends, drug, and enzyme do not exist at one place. This condition demands transport of drug through the cell membrane, and the present analysis indicated that the zone of inhibition method permitted the separation of events and provides evidence for the significance of the drug’s solubility parameter for effective interaction. It indicated that the active site in the enzyme must be polar in nature. It is obvious, because the enzyme is present in the cytoplasm aqueous environment. The two enzymes, Topoisomerase I and IV had similar protein structure, each composed of Gyr-A and Gyr-B. The protein structure exhibited polar functional groups electrostatic interaction with fluoroquinolones, the acting binding site is polar in nature, the conclusions drawn from Gram positive and Gram negative organisms remained same. Thus, a value of 11.67 H was justified for the inhibition of enzymes.
Solubility parameter of moxifloxacin HCl:
Martin et al proposed the solubility parameter of benzoic acid as 11.5H.23 Thimmasetty J et al proposed the solubility parameter of celecoxib as 11 H by a different approach.24 In this investigation an attempt was made to derive the solubility parameter value of moxifloxacin HCl using the results from different methods. The results are shown in the Table 5. From the results it can be seen that the solubility parameter of moxifloxacin HCl by group contribution method, Hoy’s method (using experimental molar volume, 287.32 cm3 /mol) is 11.48 H, from the graph of 1/MIC versus solubility parameter of solvent blends is 11.67 H, and the value derived from the graph of zone of inhibition versus solubility parameter of solvent blends is 11.67 H. As the value remains same from these methods, it can be concluded that the solubility parameter of moxifloxacin HCl is 11.67 H.
CONCLUSIONS
Hoy’s method gave identical results for estimating of the solubility parameter of moxifloxacin HCl. MIC studies revealed that solubility (giving intimate contact of this drug with microbes) was an important factor for antibacterial activity. A good correlation was obtained between the solvent rejection parameter |δ2 -δ1 | versus cumulative transport and the zone of inhibition of moxifloxacin HCl, indicating the validity of the solvent rejection principle. Therefore, microbes can be used successfully as models for the correlation of physicochemical properties such as solubility parameter of drugs, with their antibacterial activities. By integrating the entire work, it can be concluded that solubility parameter can be wisely used as an index of antibacterial activity of moxifloxacin in specific or antibiotic in general.
ACKNOWLEDGEMENT
The authors are thankful to Advance Research Wing, Rajiv Gandhi University of Health Sciences, Karnataka for its financial support in the realization of this research.
Disclosure
This research was implemented in the framework of the project “The new application of solubility parameter for predicting the antibacterial activity of moxifloxacin” (code: P001).
Supporting File
References
1. Kenneth CJ. Solubility and related properties. 3rd ed. New York:Marcel Dekker, Inc; 1986.
2. Mullins L. Some physical mechanism in narcosis.Chem Rev1954;54:289-323.
3. Khalil SA, Abdallah OA, Moustafa MA. Absorption of some barbiturates by gambusia fish and its correlation to solubility parameter. Can J Pharm Sci 1976;11:26-30.
4. Khalil SA, Martin AN. Drug transport through model membranes and its correlation with solubility parameters. J Pharm Sci 1967;56:1223- 33.
5. Adjei A, Newburger J, Stavchansky S, Martin A. Membrane solubility parameter and in situ release of theophylline. J Pharm Sci 1984;73:742- 5.
6. YueyuanY, Yunquan L, Jie C. Application of solubility parameter theory to organosolv extraction of lignin from enzymatically hydrolized cornstalks. Bioresources 2014;9(2):3417-27.
7. Mohamed K, Victoria F. Estimation of the solubility parameter of model plant surface and agrochemicals a valuable tool for understanding plant surface interactions. Theor Biol Med Model 2012;9(45):1-23.
8. Yaqi L, Maria GC, Xia L, Tim EM, Ferenc B, Richard GW, et al. Comparing and correlating solubility parameters governing the self-assembly of molecular gels using 1,3:2,4-dibenzylidene sorbitol as the gelator. Langmuir 2014;30(47):14128-42.
9. Teja K, Aleksandra D, Odon P, Rok S, Stanko S. Determination of solubility parameters of ibuprofen and ibuprofen lysinate. Molecules 2015;20:21549-68.
10. Thimmasetty J, Subrahmanyam CVS, Satheshbabu PR, Maulik MA, Viswanath BA. Solubility behaviour of pimozide in polar and nonpolar solvents, Partial solubility parameters approach. J Solution Chem 2008;37:1365-78.
11. Gaikwad ER, Khabade SS, Sutar TB, Bhat MR, Santosh AP. Three-dimensional Hansen solubility parameters as predictors of miscibility in cocrystal formation. Asian J Pharm 2017;11(4):302.
12. Manjunath K, Subrahmanyam CVS, Thimmasetty J, Nitin KS. Solubility parameter of gatifloxacin and its correlation with antibacterial activity. J Solution Chem 2012;41:381-91.
13. Indian Pharmacopoeia. 5th ed. Ghaziabad: The Indian Pharmacopoeia Commission, 2018.
14. Hoy KC. New values of the solubility parameters from vapour pressure data. J Paint Technol 1970;41:76-118.
15. Martin A, Newburger J, Adjei A.Extended Hildebrand solubility approach, Solubility of theophylline in polar binary solvents.J Pharm Sci 1980; 69(5):487-91.
16. Rathi PB. Solubility prediction of satranidazole in propylene glycol-water mixtures using extended Hildebrand solubility approach. Indian J Pharm Sci2011;73(6):670-4.
17. Martin A, Swarbrick J, Cammarata A. Physical Pharmacy. 3rd ed. Philadelphia:Lea and Febiger,1983.
18. Gradon JL.Encyclopaedia of polymer science and technology. New York: 3 Wiley: 1965.
19. Subrahmanyam CVS. Essentials of Physical Pharmacy. New Delhi, Vallabh Prakashan, 2003.
20. Ananthanarayan R, Paniker CKJ. Textbook of Microbiology. 5th ed. Chennai: Orient Longman Ltd; 1997.
21. Saeed E, Abbas S, Alireza F. Quinolones, recent structural and clinical developments. Iran J Pharm Res 2005;3:123-36.
22. Marvin JC, Martin AN. The solubility of benzoic acid in mixed solvents. J Pharm Sci 1959;49 (7):444-7.
23. Thimmasetty J, Subrahmanyam CVS, Viswanath BA, Satheshbabu PR. Solubility parameter estimation of celecoxib by current methods. Asian J Research Chem2009;2(2):188- 95.