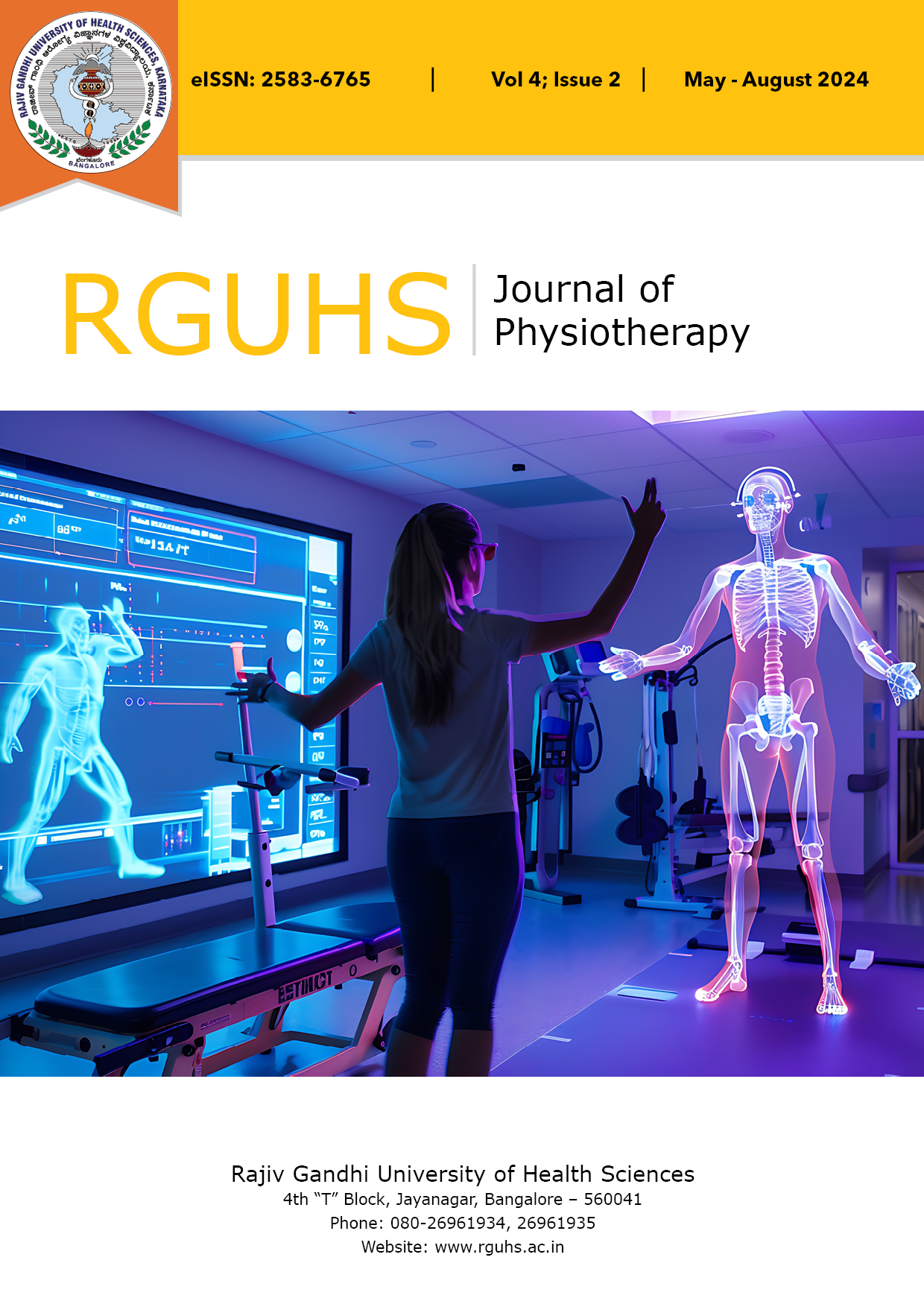
RGUHS Nat. J. Pub. Heal. Sci Vol No: 4 Issue No: 2 eISSN:
Dear Authors,
We invite you to watch this comprehensive video guide on the process of submitting your article online. This video will provide you with step-by-step instructions to ensure a smooth and successful submission.
Thank you for your attention and cooperation.
1Dr. Kavitha Raja PT, PhD, Principal, JSS College of Physiotherapy, Mysore, Karnataka, India.
*Corresponding Author:
Dr. Kavitha Raja PT, PhD, Principal, JSS College of Physiotherapy, Mysore, Karnataka, India., Email: kavitharaja_jsscpt@jssonline.org
Abstract
None
Keywords
Downloads
-
1FullTextPDF
Article
Physiotherapists recommend exercise and physical activity to enhance organ, tissue, cell, and system functions and promote each person’s health and well-being. With the advancement of our basic knowledge, our mechanistic understanding of how therapies elicit the appropriate changes also improved. For instance, we are aware that our C6 quadriplegic patients benefit from a variety of adaptive cellular responses when they acquire a strong tenodesis grip. Their wrist extensors are served by sensory and motor networks in the brain that “re-map” to support the hand muscles, boosting the neural substrate that is available to these vital muscles.
Thanks to the advancements in neuroscience, we now are aware how this adaptability is made possible via modifications in gene expression and chemical signalling cascades. We are starting to comprehend how the type and amount of exercise affects these cellular changes, owing to the studies conducted on animal models.
Physiotherapists typically see the overt functional results of management strategies, but the time to comprehend the complex molecular changes that underlie each and every functional improvement is not too far. At that point, the knowledge that physiotherapy operates on a cellular level will be universal.
Our quality care can improve significantly if the physiotherapists can apply latest important discoveries in cell biology to inform and focus our potent exercise-based therapies. Hence genetics must be made a mandatory course for admission to all physiotherapy degree programmes. A discussion on the genetic underpinnings for health-promoting effects of exercises may aid physiotherapists to raise awareness among doctors and other healthcare professionals.
Furthermore, the newest area of research in physiotherapy is called epigenetics, which studies how repeated exposure to environmental stimuli (such as emotional stress, exercise, and diet) can either promote or inhibit the expression of particular genes.
The epigenome could be one of the most successful mechanisms through which exercise realizes its broad range of benefits. Physiotherapists are well suited to add to the expanding body of knowledge in this sector because of their expertise in exercise. The major epigenetic concepts that can support and enhance the current body of scientific evidence supporting physiotherapy interventions must be emphasised in research. Physiotherapists who stay updated in this rapidly developing discipline can create an innovative movement therapy which is expertly tailored for patients of all ages, genders and activity levels.
The term “epigenetics,” which translates to “above the genome,” describes the mechanisms that regulate and direct the way genes found in the genome are expressed. Epigenetic modifications are reversible “tags” that can be added to DNA or histone molecules that link DNA to chromatin, rather than altering the DNA nucleotide base pairs itself (mutation). The epigenetic changes most extensively researched in connection to physical activity are DNA methylation and histone acetylation. Epigenetic modifications can be transmitted during cell division, whether it’s through mitosis or meiosis, despite the fact that they do not alter the genetic code.
Although there have long been intuitive connections between physical activity and good health, formal scientific research on this topic started only a few decades back. The 1970s witnessed a significant surge in scientific research on exercise, culminating in over 400,000 papers on the subject as of today. Thanks to this research, we have a better understanding of the negative correlation between physical activity and metabolic diseases, as well as the increased morbidity experienced by individuals with disabilities who frequently lack access to regular exercise. The discipline of epigenetics is about to witness a growth in understanding, comparable to the research conducted on exercise in the previous decades. The number of publications on epigenetics published annually is similar to the number of articles published about exercise in 2000.
Fewer than 600 publications, with only a handful conducted on human subjects, have examined the connections between exercise and epigenetics to date. As physiotherapists, we should pay great attention to this research because most of our movement innovations are meant to be used for an extended period of time-exactly the kind of ubiquitous “environmental” stimulation (enriched environment) that might result in lasting epigenetic adaptations. The field of physiotherapy could benefit greatly from comprehending the intricate relationship between exercise and epigenetic changes.
Extensive epidemiological research is beginning to demonstrate connections between human longevity and exercise-induced epigenetic modifications (DNA methylation). After calculating the participants’ “epigenetic age” based on their DNA methylation status, researchers examined the correlations between this discrepancy and various clinical indicators of metabolic health, dietary habits, and physical activity. Individuals with lower epigenetic age also had greater incomes, higher levels of education, higher intakes of nutritious food, higher levels of physical exercise, and better clinical markers of metabolic health. Because participants in this study were only categorised as “active” or “sedentary,” the statistical connections between epigenetic age and physical activity were weak. A comparable study done among older Swedish residents which divided physical activity into four categories found a strong, linear correlation between lifetime exercise level and blood cell methylation status, or epigenetic ageing. This relation held true even after accounting for smoking, BMI, and a number of other metabolic variables, indicating that exercise limits epigenetic ageing on its own.
These studies offer the first concrete proof that physical activity and food, when combined to safeguard metabolic health, are correlated with longevity-predicting epigenetic modifications. These studies contribute significantly to the understanding of the powerful, long-lasting effects of exercise on general health.
In order to more prospectively investigate the epigenetic modifications by which exercise may preserve health and slow ageing, some researchers have implemented exercise regimens over a limited time period. During a 6-month period, inactive men who participated in 1.8 weekly sessions of aerobic cycling showed numerous noteworthy improvements in their global metabolism, including a decreased waist/hip ratio, an increase in VO2max, a decrease in diastolic blood pressure, and an increase in HDL. These individuals’ adipose cell biopsies revealed significant changes in CpG site methylation in 21 type 2 diabetes candidate genes and 18 genes associated with obesity. Accompanying the methylation alterations were modifications in mRNA, suggesting that after undergoing exercise training, the observed epigenetic changes indeed influenced gene expression.
As a systemic stressor, exercise may cause various epigenetic modifications in various organs. Myocyte adaptations are particularly interesting because of their pivotal function in regulating metabolism and exercise. Epigenetic responses to a single session of endurance training have been documented in one study where participants showed notable modifications in histone acetylation in the vastus lateralis muscle just 60 minutes after cycling at approximately 76% VO2max. Other researchers discovered that 134 genes in the vastus lateralis, many of which have roles related to type 2 diabetes, had their methylation altered by a lengthy programme (six months) of endurance exercise (cycling and aerobics).
After three months of unilateral knee extension exercise (a within-subject control design that reduces several sources of variability), the vastus lateralis muscle’s DNA methylation and mRNA expression changed for more than 4000 gene loci. A pathway study indicated that there was a “coordinated transcriptional and epigenetic response” to exercise, with modifications in gene networks controlling structural remodelling, with glucose metabolism being particularly common. The authors proposed that epigenetic modifications may serve as a major basis for the control and maintenance of adaptations resulting from endurance exercise.
Further investigation into the connections between epigenetic changes and physical activity will help us improve the exercise recommendations for both healthy individuals and those with illness or impairment. Future applications of epigenetic knowledge to rehabilitation should take into account a number of important variables, such as variations in the tissues tested, variations in the intensity and form of exercise, and unpredictability regarding the heredity of exercise-modulated epigenetic features. Epigenetic data will probably improve our understanding of how different people react to recommended exercise in the future. Physiotherapists need to be aware of emerging epigenetic research that has potential to improve the efficacy and specificity of movement-based interventions which is our foremost treatment strategy.
Supporting File
References
- Barrès R, Yan J, Egan B, et al. Acute exercise remodels promoter methylation in human skeletal muscle. Cell Metab 2012;15:405-411.
- Kirchner H, Osler ME, Krook A, et al. Epigenetic flexibility in metabolic regulation: disease cause and prevention? Trends Cell Biol 2013;23:203-209.
- McGee SL, Fairlie E, Garnham AP, et al. Exercise-induced histone modifications in human skeletal muscle. J Physiol 2009;587: 5951-5958.
- Rasmussen M, Zierath JR, Barrès R. Dynamic epigenetic responses to muscle contraction. Drug Discov Today 2014;232;19:1010-1014.
- Denham J, Marques FZ, O’Brien BJ, et al. Exercise: putting action into our epigenome. Sports Med 2014;44:189-209.
- Berger SL, Kouzarides T, Shiekhattar R, et al. An operational definition of epigenetics. Genes Dev 2009;23:781-783.
- Eads CA, Danenberg KD, Kawakami K, et al. MethyLight: a high-throughput assay to measure DNA methylation. Nucleic Acids Res 2000;28:E32.
- Chatterjee A, Rodger EJ, Morison IM, et al. Tools and strategies for analysis of genomewide and gene-specific DNA methylation patterns. Methods Mol Biol 2017;1537: 249-277.
- Fazzari MJ, Greally JM. Introduction to epigenomics and epigenome-wide analysis. Methods Mol Biol 2010;620:243-265.
- Abel JL, Rissman EF. Running-induced epigenetic and gene expression changes in the adolescent brain. Int J Dev Neurosci 2013;31:382-390.
- Tsai HW, Grant PA, Rissman EF. Sex differences in histone modifications in the neonatal mouse brain. Epigenetics 2009;4:47-53.
- Gomez-Pinilla F, Zhuang Y, Feng J, et al. Exercise impacts brain-derived neurotrophic factor plasticity by engaging mechanisms of epigenetic regulation. Eur J Neurosci 2011; 33:383-390.