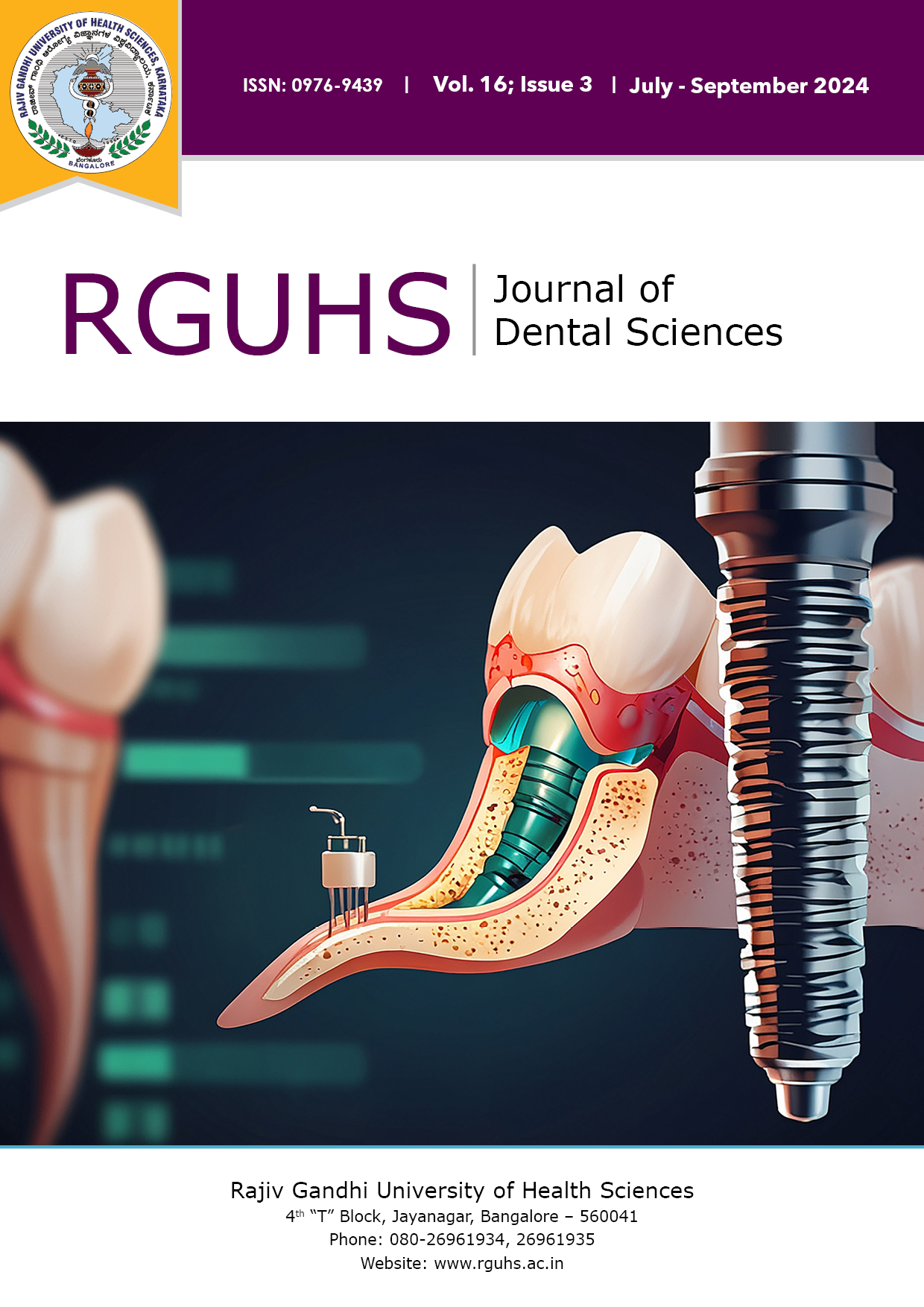
RGUHS Nat. J. Pub. Heal. Sci Vol No: 16 Issue No: 3 pISSN:
Dear Authors,
We invite you to watch this comprehensive video guide on the process of submitting your article online. This video will provide you with step-by-step instructions to ensure a smooth and successful submission.
Thank you for your attention and cooperation.
Kamath Apoorva Shrinivasa
Senior Lecturer, Department of Orthodontics and Dentofacial Orthopaedics, Srinivas Institute of Dental Sciences, Mangalore-574146, Karnataka, India.
*Corresponding author:
Dr. Kamath Apoorva Shrinivasa, Senior Lecturer, Department of Orthodontics and Dentofacial Orthopaedics, Srinivas Institute of Dental Sciences, Affiliated to Rajiv Gandhi University of Health Sciences, Karnataka. Mukka, Mangalore-574146, Karnataka, India.
Received date: June 6, 2021; Accepted date: June 24, 2021; Published date: June 30, 2021

Abstract
The science of tissue engineering is a promising one to a craniofacial biologist with a plethora of research studies being conducted over the years. Stem cells (SCs) along with scaffolds and biological stimulants have been at the epicenter of such studies. The field has also gained importance due to prevalent drawbacks with organ transplantation, such as shortage in the number of donors coupled with other complications associated with the procedure. Moreover, it finds its applications in congenital defects as well. Several electronic databases were thoroughly researched including the Directory of Open Access Journals (DOAJ), Google scholar, Digital Dissertations and meta Register of Controlled Trials for relevant data. References and citations of articles and existing reviews were also researched. The search was confined to articles in English, published till March 2021. Casecontrol, cross-sectional, retrospective and prospective studies, systematic reviews and meta-analysis were included in this article. A hand search of the reference lists of the included articles was also carried out to include missed out articles. Here, the aim was to provide a comprehensive review of tissue engineering in general and its application in the craniomandibular region in particular to some of the potential futuristic novel methods which are being developed.
Keywords
Downloads
-
1FullTextPDF
Article
Introduction
The craniomandibular system is a complex system composed of bones and cartilage with the conterminous soft tissues, neural innervation and vasculature. The balance and harmony of this system may be altered due to craniofacial anomalies, trauma, progressive skeletal diseases or tumor resection. Treatment of such defects entails either replacement or regeneration of the diseased structures to restore the hosts’ craniomandibular system to its state of physiologic equipoise.1-3
Repair and reconstruction of large craniofacial defects however, is a formidable task for the oral and maxillofacial surgeons. Clinical practice over the years aimed to treat such defects by a combination of autologous grafts and surgical interventions.4 Structures of the craniomandibular system are largely derived from mesenchymal cells. A cell population called as mesenchymal stem cells are derivatives of the mesenchymal cells and have been isolated from the dental pulp, the periodontium and the primary tooth. It is this cell population which may be delivered to the site of interest or recruited internally, that forms the biologic basis of tissue engineering in the craniomandibular system.5
This review gives an insight of the tissue engineering procedures that have been developed for de-novo regeneration of the craniomandibular structures and also discusses the recent advances in this science which have set a stage for its foreseeable future.
Methodology
Several electronic databases were thoroughly researched including the Directory of Open Access Journals (DOAJ), Google scholar, Digital Dissertations and meta Register of Controlled Trials for relevant data. The search was confined to articles in English, published till March 2021. Case-control, cross-sectional, retrospective and prospective studies, systematic reviews and metaanalysis were included in this article. A hand search of the reference lists of the included articles was also carried out to include missed out articles.
Doctrine of Tissue Engineering
The science of tissue engineering in dentistry was contrived by Nyman and colleagues in 1982, who regenerated the periodontal ligament by a procedure called Guided Tissue Regeneration (GTR).6 Though craniomandibular tissue engineering is yet to achieve its full potential owing to the challenges posed, it is a promising science for the future of the specialty. The principles of craniomandibular tissue engineering as elsewhere encompass replacement of functionally diseased, aging or lost tissues by in vitro tissue analogs in which responsive progenitor cells or stem cells are integrated into a framework of extracellular matrix of signaling molecules inducing morphogenesis. 6-9 (Figure 1)
Stem cells (SCs) defined as cells not regimented or restricted to a fixed number of divisions mitotically can be classified broadly as adult or postnatal and embryonic. Adult SCs having a more regulated differentiation potential compared to their embryonic counterparts have been at the epicenter of tissue engineering procedures.10,11 Induced pluripotent SCs are adult SCs expressing embryonic SC like phenotype and possess an ability to differentiate into any desired cell type, a property also referred to as plasticity.12 (Table 1)
The framework/scaffold laden with cells need to be stimulated to enhance the activity of SCs and promote their differentiation to the desired cell type. This is essentially achieved through two types of signaling molecules i.e. growth factors and immobilized extracellular molecule peptides. These bioactive peptides can also be delivered to the desired scaffold/framework by transport of secreting cells to the framework.13,14 Families of proteins such as Bone Morphogenetic Proteins (BMPs), Hedgehog proteins, Wingless- and int-related proteins and Fibroblast Growth Factors (FGFs) control the process of tissue morphogenesis.9 Alternatively, the cells can also be stimulated by exposing them to a myriad of biologically potent chemical or mechanical stimuli.15 (Table 2)
The scaffolds used in the process of tissue engineering today range from a plethora of synthetic and natural polymers to ceramics. The extracellular framework is biomimetic and plays a crucial role in new tissue formation by the agents inducing morphogenesis.9 Over the years, their role in the process has seen a shift from a simple interaction with the body to a more significant one of directing the body’s biological systems in achieving the objectives of tissue engineering.15 (Figure 2)
Applications in the Craniomandibular System (Figure 3)
I. Craniofacial orthopedics
Development of the craniofacial skeleton involves extremely well-coordinated and exacting processes. SCs are increasingly being used to therapeutically reconstruct large craniofacial defects. Adipose derived adult SCs can heal surgically induced defects in the mouse craniofacial skeleton when seeded on apatite covered poly glycolic acid (PGA) scaffolds by intramembranous bone deposition without necessitating any exogenous growth factor addition or manipulation or genes.16 Alternately, isolated autologous bone marrow stem cells from rat sutures have been seeded on collagen scaffolds co-seeded with microspheres of encapsulated Transforming Growth Factor-β3 (TGF-β3 ). This combination prevented bone formation and hence re-synostosis in contrast to a placebo group lacking the TGF-β3 controlled release spheres. TGF-β3 has been found to be an inhibitor of ossification. Thus, re-osteogenesis can also be minimized by a biologically engineered tissue model composed of soft tissue-bone interface.17
II. Repair of cleft of the alveolus
Grafting of the cleft alveolus post expansion is vital since it restores arch continuity, ensures sealing of the oronasal fistula, provides path for tooth eruption and also braces the alar base of the affected side. While the standard of care for such a defect is an autogenous bone graft, tissue engineering has been explored extensively over the past four decades.18 The popularity of this procedure draws from the fact that it is minimally invasive and has shown good healing of the adjacent soft tissues.19 Apropos to grafting of the defect, tissue engineering strategies can be broadly categorized into those enhancing the quality of the autogenous bone graft, those expanding graft volume in cases where adequate volume of autogenous bone could not be harvested and those using synthetic grafts in its entirety. Synthetic grafts commonly employ recombinant human BMP-2 or calcium phosphate in conjunction with SCs on a scaffold, the types of which have risen greatly over time.18 A reduction in the morbidity and an improvement in the healing profile with BMP-2 in the repair of cleft alveolus defect is seen in older individuals.20
III. Reconstruction of nasal defects
Defects of the nose often lead to functional and esthetic problems. Traditionally, the mainstay of reconstruction of such defects has been autologous grafts or local flaps. However, because of the inherent limitation of these procedures, tissue engineered constructs are being explored for their potential applications.21 Autologous transplants of the nasal cartilage have also been used after seeding chondrocytes on a pre-shaped bio-degradable mesh.22 Scaffolds of marine collagen which are used for nasal septal perforation possess excellent features for tissue engineering of the nasal cartilage with significantly less number of perforations in comparison to no intervention.23 However, nasal reconstruction of a full-thickness defect with neogenesis of the mucosal, cartilaginous and soft tissues of the nose has not been so far demonstrated.21
IV. Reconstruction of otic defects
The auricular cartilage in general and repair of perforation of the tympanic membrane has been the focus of tissue engineering techniques for the auricle, with the first attempt of such a reconstruction procedure being carried out by Dr. R. Tanzer. Its intricacies were defined by Dr. B. Brent who gave a four-stage procedure for the same. The most frequently performed technique currently is the twostage procedure developed by Drs. S. Nagata and F. Firmin.21,24
Auricular, articular and nasoseptal cartilages retain architecture, expression of genetic characteristics and composition of matrix which is comparable to the normal ear cartilage. Costal chondrocyte due to mineralization of the extracellular matrix were found to be inappropriate for ear cartilage reconstruction.25
V. Reconstruction of ocular defects
Tissue engineered substitutes for the ocular tissues have come a long way over the years. However, they need further refinement to make them clinically suitable. Damaged and diseased corneas have traditionally been replaced entirely or partially by donated corneal tissues. However, shortages in donors, risk of infection secondary to the surgery and a possibility of rejection of the donor tissue demands alternative avenues to the problem associated with grafting. The three layers of the cornea namely epithelium, stroma and endothelium have successfully been engineered.26
Ocular surface reconstruction aims at restoring the integrity of two highly distinct and specialized tissues i.e. the corneal and conjunctival epithelia. Keratoepithelioplasty employs corneal lenticules to generate corneal epithelial cells. Corneal limbal stem cells have vast implications on the development of keratoepithelioplasty. Amniotic membrane transplantation has been used for inhibition of pathological scarring in ocular surface reconstruction which has subsequently been used with limbal allografts for severe ocular surface defects.27
The lacrimal gland secretions form a part of the tear film that provides lubrication and protection to the ocular surface. Baum et al envisioned the construction of an artificial fluid secreting device via bio-engineering. Intact acini of the lacrimal gland when cultured maintain their histologic characteristics and physiologic response to stimuli.28
VI. Reconstruction of the Temporomandibular joint (TMJ)
Neogenesis of the TMJ holds a challenge from a tissue engineering perspective due to the myriad nature of tissue types that form the joint space. Restoration of the physical, chemical and biological characteristics of the same defines a successful reconstruct. The cells used for the process differ according to the type of the tissue they aim to replace.
Mandibular condyle reconstruction has traditionally been attempted from native adult cartilage cells of the condyle. They may be harvested, minced and isolated following collagenase treatment or the cells may be intentionally allowed to migrate onto surgical sponges from the fibrous zone of the condyle.29 Characterization of the superior joint space has been inadequate so forth.TMJ disc constructs show superior matching to the TMJ disc compared to other musculo-skeletal soft tissue constructs. Cell sources for the same vary from TMJ discal cells, articular and costal chondrocytes.30
VII.Dental tissue engineering
Teeth are indispensable for the optimum functioning and structural balance of the craniomandibular system. Principles of tissue engineering and regenerative surgical techniques in conjugation with conventional orthodontic mechanotherapy has shortened treatment duration in addition to increasing the scope of the therapy. This procedure largely increases the rate of tissue turnover by two to three folds while reducing the side effects such as relapse and insufficient alveolar bone levels.31
Restoration of a lost tooth structure or whole tooth, regeneration of its root and contiguous PDL structures is also being carried out. Periapical follicular SCs from the developing root apex of the third molars have been successfully used as a potential source of cells for periodontal and bio-root engineering.8 Loss of tooth vitality due to the sequelae of caries necessitates root canal therapy which compromises the ability of the tooth to fight bacterial insult while rendering it brittle. Pulp regeneration has been attempted and reported in animal models. However, challenges such as neovascularization, de-novo innervation and resolution of the inflammation of the initial pathology have limited its translation into full clinical application.8,32
VIII.Distraction osteogenesis
It is an endogenous form of tissue engineering employed to treat large craniofacial growth defects or deficiencies. Since its inception for distraction of deficient mandibles in the 1990s, stimulation of new bone formation has been achieved mainly due to the tensile strain and the ensuing surgical trauma.33 Adjuncts to facilitate the process include low intensity pulsed ultrasound, electrical stimulation, besides a host of growth factors and pharmacological agents.
The goal of tissue engineering in distraction osteogenesis is to abbreviate treatment duration, i.e. faster rate of distraction and shorter durations of consolidation. However, only few studies have explored this aspect.34 Hence it could not be conclusively said whether it shortens treatment duration and a refinement of the animal models, stem cell transplant and distraction protocols are necessary and need thorough investigation.35
IX. Rapid maxillary expansion
The maintenance of the results of maxillary expansion is as crucial as the expansion process per se, to prevent relapse and achieve a stability of the results obtained. Local application of mesenchymal SCs derived from the bone marrow into the premaxillary suture after activation of expansion yielded increased vasculature and osteoblasts in the site, thus accelerating neo-osteogenesis and shortening the reinforcement and consolidation period post expansion.36
Orthodontic expansion on a graft comprising of bone marrow mesenchymal SCs on a β-tricalcium phosphate scaffold showed tissue engineering with bone and rapid maxillary expansion produced bony trabeculae in greater abundance and in an increased area. However, no significant differences were noted between bone construct formed from bone marrow mesenchymal SCs with β-tricalcium phosphate and autogenous grafts.37
Future Trends in Tissue Engineering
Nanotechnology is making its imprints in the field of tissue engineering offering greater versatility in designing the scaffolds. Applications of nanotechnology have been extended to the field of craniofacial defect repair. Nanohydroxyapatites, which are alloplastic counterparts of the inorganic hydroxyapatite crystals of bone have been used as a composite with micro betacalcium phosphate to enhance its binding and retention to intrabony defects.38 Nanoparticles along with nanotubes and/or nanofibres have shown to enhance mechanical properties of the scaffolds with better attachment of cells, thus facilitating tissue engineering.13 Biomimetic scaffold comprising peptide nanofibres of 6-nanometre diameter can be used for regeneration of the pulpal tissues. In addition to encapsulation of the pulpal stem cells, the nanofibres encapsulate growth factors such as vascular endothelial growth factor and TGF-β1 . 39
Three-dimensional printing derives its importance in tissue engineering due to controlled and repeatable scaffolds produced. Three-dimensional printing techniques currently available include laser sintering, stereolithography, extrusion printing and powder bed inkjet printing.40 With the advent of 3D printing in the replacement of the complicated template fabrication procedure such as in the calvaria, the procedure has become more specific.13
Evolution of tissue engineering has also seen the emergence of scaffold free techniques to offset the complications of scaffold compatibility with the host. Autologous cells are grown into continuous sheets with their extracellular matrix, which is known as “cell sheet technology”.41 It exploits the ability of cells to produce extracellular matrix for creating three dimensional tissues and was popularized following the pioneering work of Holtfreter and Mascona. Steinberg examined the ability of isolated cells to re-associate into tissues and proposed the differential adhesion hypothesis (DAH). This hypothesis was put forth to display the reaggregation potential of isolated cells.42
Limitations
Tissue engineering as a science though very promising, is still yet to be studied on a long-term basis. It needs skill and knowledge for proper application and is quite uneconomical at present. Non-uniform results, for example implantation of osteo-induced stem cells into rabbit calvarial defects have not improved bone healing significantly in comparison to controls.43
Conclusion
The branch of regenerative medicine through tissue engineering has opened newer avenues for craniomandibular rehabilitation. Success in animal models has not adequately translate into full clinical application in humans. Long term studies to evaluate the predictability of these methods is the need of the hour. Despite of these shortcomings, the science holds tremendous scope and needs further research.
Conflict of Interest
None.
Supporting File
References
- Petrovic V, Zivkovic P, Petrovic D et al. Craniofacial bone tissue engineering. Oral Surg Oral Med Oral Pathol Oral Radiol 2012;114(3):e1-9.
- Herring SW, Ochareon P. Bone–special problems of the craniofacial region. Orthodontics & Craniofacial Research 2005;8(3):174-82.
- Shakya AK, Kandalam U. Three-dimensional macroporous materials for tissue engineering of craniofacial bone. British Journal of Oral and Maxillofacial Surgery 2017;55(9):875-91.
- Yanaga H, Yanaga K, Imai K et al. Clinical application of cultured autologous human auricular chondrocytes with autologous serum for craniofacial or nasal augmentation and repair. Plastic and reconstructive surgery 2006;117(6):2019-30.
- Mao JJ, Giannobile WV, Helms JA et al. Craniofacial tissue engineering by stem cells. Journal of Dental Research 2006;85(11):966-79.
- Nyman S, Lindhe J, Karring T et al. New attachment following surgical treatment of human periodontal disease. Journal of Clinical Periodontology 1982; 9(4):290-6.
- Krishnan V, Davidovitch ZE, editors. Integrated clinical orthodontics. John Wiley & Sons; 2012.
- Horst OV, Chavez MG, Jheon AH et al. Stem cell and biomaterials research in dental tissue engineering and regeneration. Dental Clinics 2012;56(3):495- 520.
- Nakashima M, Reddi AH. The application of bone morphogenetic proteins to dental tissue engineering. Nature biotechnology. 2003;21(9):1025-32.
- Caplan AI. Mesenchymal stem cells. Journal of orthopaedic research. 1991;9(5):641-50.
- Ivanovski S, Gronthos S, Shi S et al. Stem cells in the periodontal ligament. Oral diseases. 2006;12(4):358-63.
- Yen AH, Yelick PC. Dental tissue regeneration–a mini-review. Gerontology. 2011;57(1):85-94.
- Borrelli MR, Hu MS, Longaker MT et al. Tissue engineering and regenerative medicine in craniofacial reconstruction and facial aesthetics. The Journal of craniofacial surgery. 2020;31(1):15.
- Boontheekul T, Mooney DJ. Protein-based signalling systems in tissue engineering. Current opinion in biotechnology. 2003;14(5):559-65.
- O’brien FJ. Biomaterials and scaffolds for tissue engineering. Materials today. 2011;14(3):88-95.
- Cowan CM, Shi YY, Aalami OO et al. Adiposederived adult stromal cells heal critical-size mouse calvarial defects. Nature biotechnology. 2004;22(5):560-7.
- Moioli EK, Clark PA, Sumner DR, et al. Autologous stem cell regeneration in craniosynostosis. Bone. 2008;42(2):332-40.
- Janssen NG, Weijs WL, Koole R et al. Tissue engineering strategies for alveolar cleft reconstruction: a systematic review of the literature. Clinical Oral Investigations. 2014;18(1):219-26.
- Behnia H, Khojasteh A, Soleimani M et al. Secondary repair of alveolar clefts using human mesenchymal stem cells. Oral Surgery, Oral Medicine, Oral Pathology, Oral Radiology and Endodontology. 2009;108(2):e1-6.
- Dickinson BP, Ashley RK, Wasson KL et al. Reduced morbidity and improved healing with bone morphogenic protein-2 in older patients with alveolar cleft defects. Plastic and reconstructive surgery. 2008;121(1):209-17.
- Niermeyer WL, Rodman C, Li MM, et al. Tissue engineering applications in otolaryngology—The state of translation. Laryngoscope Investigative Otolaryngology. 2020;5(4):630-48.
- Naumann A, Rotter N, Bujia J, et al. Tissue engineering of autologous cartilage transplants for rhinology. American Journal of Rhinology. 1998;12(1):59-64.
- Bermueller C, Schwarz S, Elsaesser AF et al. Marine collagen scaffolds for nasal cartilage repair: prevention of nasal septal perforations in a new orthotopic rat model using tissue engineering techniques. Tissue Engineering Part A. 2013;19(19- 20):2201-14.
- Sterodimas A, de Faria J, Correa WE et al. Tissue engineering and auricular reconstruction: a review. Journal of Plastic, Reconstructive & Aesthetic Surgery. 2009;62(4):447-52.
- Kusuhara H, Isogai N, Enjo M et al. Tissue engineering a model for the human ear: assessment of size, shape, morphology, and gene expression following seeding of different chondrocytes. Wound Repair and Regeneration. 2009;17(1):136-46.
- Nakamura T, Inatomi T, Sotozono C et al. Ocular surface reconstruction using stem cell and tissue engineering. Progress in retinal and eye research. 2016;51:187-207.
- Selvam S, Thomas PB, Yiu SC. Tissue engineering: current and future approaches to ocular surface reconstruction. The ocular surface. 2006;4(3):120- 36.
- Takigawa M, Okada M, Takano T et al. Studies on chondrocytes from mandibular condylar cartilage, nasal septal cartilage, and spheno-occipital synchondrosis in culture. I. Morphology, growth, glycosaminoglycan synthesis, and responsiveness to bovine parathyroid hormone (1-34). Journal of dental research. 1984;63(1):19-22.
- Thomas M, Grande D, Haug RH. Development of an in vitro temporomandibular joint cartilage analog. Journal of oral and maxillofacial surgery. 1991;49(8):854-6.
- Murphy MK, Mac Barb RF, Wong ME et al. Temporomandibular joint disorders: A review of etiology, clinical management, and tissue engineering strategies. The International journal of oral & maxillofacial implants. 2013;28(6):e393.
- Adusumilli S, Yalamanchi L, Yalamanchili PS. Periodontally accelerated osteogenic orthodontics: An interdisciplinary approach for faster orthodontic therapy. Journal of pharmacy & bioallied sciences. 2014;6(Suppl 1):S2.
- Kasper FK. Tissue Engineering for Orthodontists: The Transforming Science Simplified. In Seminars in Orthodontics 2017;23(4);355-65. WB Saunders.
- McCarthy JG, Schreiber J, Karp N et al. Lengthening the human mandible by gradual distraction. Plast reconstr surg. 1992;89(1):1-8.
- Long J, Li P, Du HM et al. Effects of bone morphogenetic protein 2 gene therapy on new bone formation during mandibular distraction osteogenesis at rapid rate in rabbits. Oral Surgery, Oral Medicine, Oral Pathology, Oral Radiology, and Endodontology. 2011;112(1):50-7.
- Tee BC, Sun Z. Mandibular distraction osteogenesis assisted by cell-based tissue engineering: a systematic review. Orthodontics & craniofacial research. 2015;18:39-49.
- Ekizer A, Yalvac ME, Uysal T et al. Bone marrow mesenchymal stem cells enhance bone formation in orthodontically expanded maxillae in rats. The Angle Orthodontist. 2015;85(3):394-9.
- Huang J, Tian B, Chu F et al. Rapid maxillary expansion in alveolar cleft repaired with a tissueengineered bone in a canine model. Journal of the mechanical behavior of biomedical materials. 2015;48:86-99.
- Tao O, Wu DT, Pham HM et al. Nanomaterials in craniofacial tissue regeneration: A review. Applied Sciences. 2019;9(2):317.
- Galler KM, Hartgerink JD, Cavender AC et al. A customized self-assembling peptide hydrogel for dental pulp tissue engineering. Tissue Engineering Part A. 2012;18(1-2):176-84.
- Rider P, Kačarević ŽP, Alkildani S et al. Bioprinting of tissue engineering scaffolds. Journal of tissue engineering. 2018;9:2041731418802090.
- Elloumi-Hannachi I, Yamato M, Okano T. Cell sheet engineering: a unique nanotechnology for scaffold-free tissue reconstruction with clinical applications in regenerative medicine. Journal of internal medicine. 2010;267(1):54-70.
- Czajka CA, Mehesz AN, Trusk TC et al. Scaffoldfree tissue engineering: organization of the tissue cytoskeleton and its effects on tissue shape. Annals of biomedical engineering. 2014;42(5):1049-61.
- Dudas JR, Marra KG, Cooper GM et al. The osteogenic potential of adiposederived stem cells for the repair of rabbit calvarial defects. Annals of Plastic Surgery 2006;56:5438.
- Lee SM, Zhang Q, Le AD. Dental stem cells: sources and potential applications. Current Oral Health Reports. 2014;1(1):34-42.
- Zhang Q, Shi S, Liu Y, et al. Mesenchymal stem cells derived from human gingiva are capable of immunomodulatory functions and ameliorate inflammation-related tissue destruction in experimental colitis. J Immunol. 2009;183(12):7787–98.