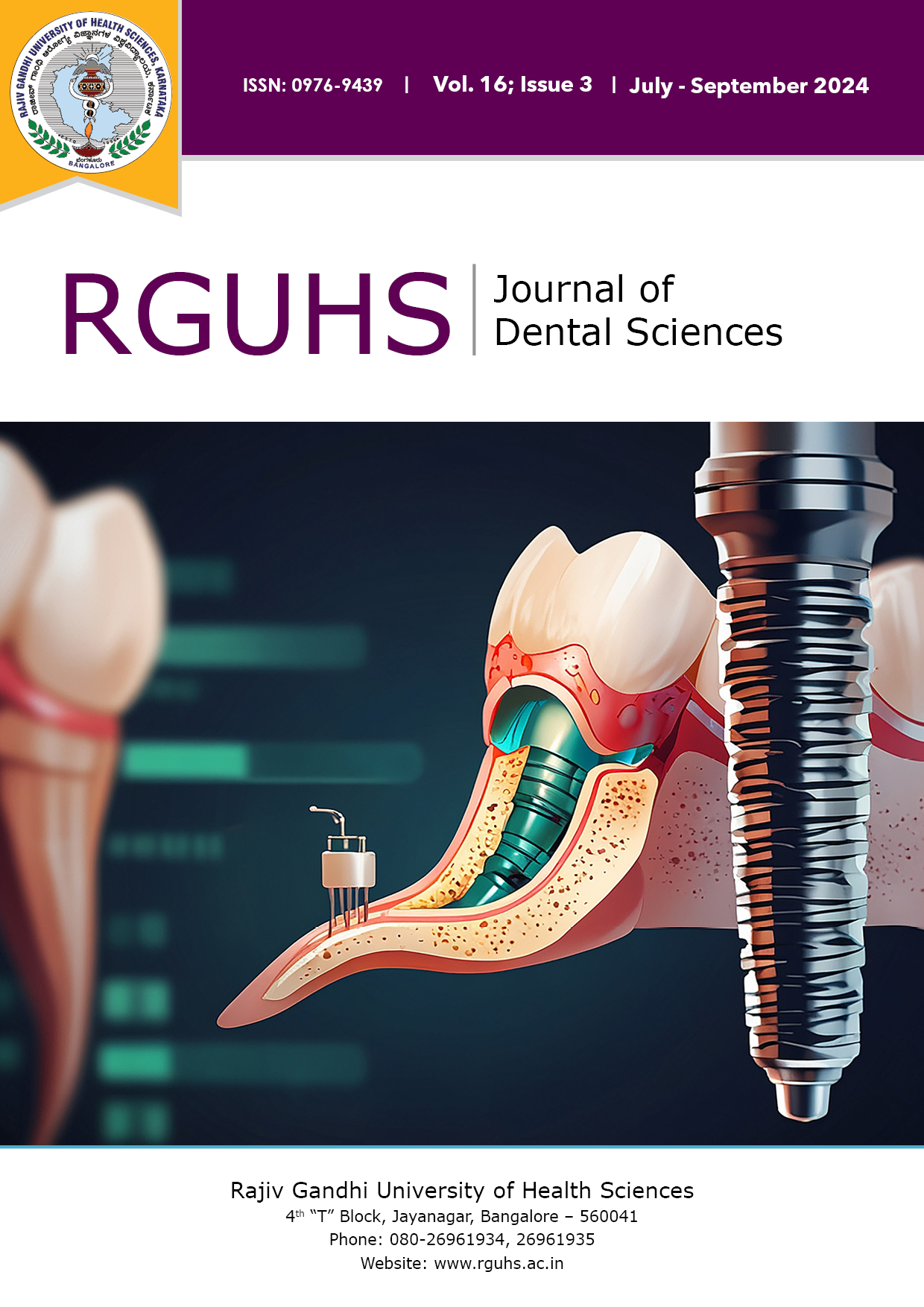
RGUHS Nat. J. Pub. Heal. Sci Vol No: 16 Issue No: 3 pISSN:
Dear Authors,
We invite you to watch this comprehensive video guide on the process of submitting your article online. This video will provide you with step-by-step instructions to ensure a smooth and successful submission.
Thank you for your attention and cooperation.
1Dr. Bhavya B, Reader, Department of Periodontics, M.S. Ramaiah Dental College and Hospital, M.S.R.I.T Post, M.S.R Nagar, Bangalore- 560054, Karnataka, India.
2Reader, Department of Periodontics, Sri Rajiv Gandhi College of Dental Sciences, Bangalore, Karnataka, India.
3Postgraduate student, Department of Periodontics, M.S. Ramaiah Dental College and Hospital, Bangalore, Karnataka, India.
4Reader, Department of Prosthodontics, D.A.P.M.R.V. Dental College and Hospital, Bangalore, Karnataka, India
*Corresponding Author:
Dr. Bhavya B, Reader, Department of Periodontics, M.S. Ramaiah Dental College and Hospital, M.S.R.I.T Post, M.S.R Nagar, Bangalore- 560054, Karnataka, India., Email: bhavyashetty@rediffmail.com
Abstract
None
Keywords
Downloads
-
1FullTextPDF
Article
INTRODUCTION
Subgingival calculus surfaces are usually covered with a layer of unmineralized and metabolically active bacteria. The essential component of conventional periodontal therapy is the effective removal of these bacterial deposits from the root surface, along with calculus deposits, in order to create a biologically compatible root surface1 .
Subgingival root debridement currently comprises the systematic treatment of all diseased root surfaces using handsonic and ⁄ or ultrasonic instruments, followed by tactile control with a periodontal probe, explorer or curette, until the root surface feels smooth and clean. However, traditional tactile perception of the subgingival environment without visible access before and after treatment frequently lacks sensitivity, specificity and reproducibility, and thus may lead to the unwanted removal of cementum, residual calculus, or both2 .
The correct evaluation of a cleaned surface is key to enable thorough and substance- sparing debridement. To support the clinician's decision to either stop or continue therapy, the past few years have witnessed the development of several calculus-detection techniques based on different technologies, which would minimize loss of healthy tooth structure, decrease chair-side time and increase efficiency in scaling. The aim of this article was to provide a critical review of various calculus detection devices based on currently available clinical and experimental data.
DETECTION-ONLY SYSTEMS
Fiberoptic endoscopy-based technology
The idea to modify a medical endoscope for periodontal use has, to date, been realized in only one device ( Perioscopy; Perioscopy Inc., Oakland, CA, USA), which was introduced in the year 2000. Perioscopy is a minimally invasive miniature periodontal endoscope which is inserted into the periodontal pocket and permits visualization of the root surface within the subgingival environment at magnifications of 24–48. The system consists of a 1 mm, 10,000-pixel fiberoptic bundle surrounded by multiple illumination fibers, a light source, an irrigation system and a liquid crystal display monitor. Clinicians can observe the subgingival root surface, tooth structure and residual calculus in real time. The magnified images can be viewed on the monitor in real time, and images and videos can be captured and saved in computer files. The endoscope may help to identify, locate and treat calculus spots during instrumentation of residual calculus at the time of, or after, scaling3 .
In the first clinical study, non-responding periodontal sites (n = 44; probing depth 5–8 mm) were treated by subgingival root debridement with or without use of the dental endoscope. No significant changes regarding pocket depth reduction were reported in either group, 1 and 3 months after treatment, compared with baseline4 .
A randomized, controlled, clinical study evaluated the percentage of residual calculus after tooth extraction in 100 single-rooted teeth of 15 patients. The teeth were treated by hand- and ultrasonic instruments until the root surface was found to be clean, as assessed by either an explorer or the periodontal endoscope. After extraction, a higher percentage of residual calculus covering the root surface was detected microscopically in the explorer group than in the endoscope group (D = 2.1%)5 .
Spectro-optical technology
The spectro-optical approach to calculus detection uses a light-emitting diode and fiberoptic technology, and is currently used by only one device, the DetecTar (Dentsply Professional, York, PA, USA). The characteristic spectral signature of subgingival calculus, which is caused by absorption, reflection and diffraction when irradiated by red light, is sensed by an optical fiber and converted into an electrical signal that is analyzed by a computer-processed algorithm. The DetecTar device comes as a portable cordless handpiece with a curved periodontal probe that has millimeter markings to measure pocket depths. Without any tactile pressure, the subgingival root surface can be scanned by the instrument. As soon as calculus is detected, the operator receives the information on calculus localization by audible and luminous signals6 .
A recent clinical study sought to determine the utility of the spectro-optical technology for subgingival calculus removal. A total of 44 teeth (176 surfaces) were included in the study. In an untreated control group, a total of 96 untreated surfaces were scanned in vivo using the DetecTar. In the treatment group, treatment was initiated upon obtaining positive signals from the spectro-optical device, and the treatment was continued until no signal was elicited. Clinical calculus findings were documented by visual and microscopic examination after tooth extraction. The control group showed a sensitivity of only 79.4% and a specificity of 95.1%. Of 58 tooth surfaces that initially showed calculus and which were consequently treated until they tested negative for calculus, 10 (17%) remained partly covered with calculus, whereas 48 (83%) were completely calculus-free. Nevertheless, nine (41%) of the 22 surfaces that were initially identified as calculus-free (and therefore untreated) did, in fact, harbor calculus 7.
Autofluorescence-based technology
The ability of calculus to emit fluorescent light following irradiation with light of a certain wavelength enables the detection of calculus, and several in vitro studies have examined the autofluorescence of dental root surfaces and calculus. Oral microorganisms and their metabolites (metalfree porphyrins, metalloporphyrins and other chromatophores) are assumed to contain the fluorophores that are emitted from dental calculus and from carious lesions. Several distinct fluorescence bands between 570 and 730 nm were identified on calculus specimens, which could be elicited with light of wavelength 400–420 nm, but could not be found on clean root surfaces. In order to differentiate calculus from the healthy tooth surface, a fluorescence-ratio method based on autofluorescence induced by a blue light-emitting diode of 405 nm has been developed. Calculus and healthy tooth surfaces exposed to light wavelengths of 487 and 628–685 nm were used to create a calculus parameter, R, which was selected to define a relationship between the integrated intensities specific for calculus and for healthy teeth in the 628 to 685- and the 477 to 497-nm wavelength regions, respectively. A cut-off threshold of R = 0.2 was able to distinguish dental calculus from healthy teeth with 100% sensitivity and 100% specificity under various experimental conditions In vitro.
A diagnostic instrument, based on different autofluorescence intensities after stimulation with red light, claims to distinguish healthy from carious tooth substance (Diagnodent; KaVo, Biberach, Germany). An indium gallium arsenide phosphate (InGaAsP)-based red laser diode (< 1 mW) sends light with a wavelength of 655 nm through an optical fiber onto the root surface, which is then induced to fluoresce. The emitted fluorescent light returning from the tooth tissue is captured by surrounding optical fibers and transmitted to an integrated photo diode, which serves as the fluorescence detector. Optical effects caused by reflected light and ambient light are eliminated by a band-pass filter and modulation of the fluorescent light, respectively. The device was primarily developed for caries diagnosis and launched as a stand-alone device about 10 years ago. Based on a multitude of clinical studies, it is considered to be a reliable caries detector on occlusal and smooth surfaces, showing high levels of sensitivity (92.1%) and specificity (100%), a high level of reproducibility (kappa value: in vitro, 0.9; in vivo, 0.9) and a good interexaminer and intra-examiner agreement8 .
The autofluorescence-based device for calculus detection has been evaluated only in in vitro studies so far, with any patient-derived clinical evidence lacking. Surfaces of extracted periodontally involved teeth, which were partly covered with calculus and moistened with saline solution or blood, were scanned using the device. The fluorescence signals detected were compared with visual and histological findings. The presence of calculus was significantly correlated with a higher intensity of fluorescence 9.
COMBINED DETECTION TREATMENT DEVICES
Ultrasonic technology
Ultrasonic calculus-detection technology is based on a conventional piezo-driven ultrasonic scaler and is similar to the way that one might tap on the rim of a glass with a spoon to identify cracks acoustically10 . An insert at a conventional dental ultrasound scaler receives short, weak impulses with a frequency of about 50 Hz, which make the insert's distal tip oscillate at a frequency that is dependent upon the surface characteristics. The oscillations are conducted into the piezoceramic discs, which transform the oscillations into voltage. The voltage level represents the intensity of the tip oscillation, while the frequency stays the same. The overall signal, consisting of both the impulse stimulus and the impulse response, is evaluated using a computerized system, thereby generating information about a given surface characteristic. The ultrasonic device currently available (Perioscan; Sirona, Bensheim, Germany) provides a detection mode to discriminate between calculus deposits and clean roots, along with a treatment mode that allows conventional ultrasonic treatment at different power levels. When the ultrasonic tip touches the tooth surface, the detection results are indicated by a light signal integrated both in the handpiece and in a display of the table unit (green indicates cementum and blue indicates calculus). When calculus is detected, an additional acoustic signal sounds. The detection mode is only activated when no scaling treatment is performed. The detection and treatment modes can be used successively on the surface of the same tooth. If calculus deposits are found, the root surface can be treated with a higher power setting, whereas in the absence of calculus (thus requiring the systematic removal only of biofilm), instrumentation can be performed at a lower power setting11 .
The only available study involving the clinical application of this ultrasound tool tested the accuracy by which calculus was detected. In vivo calculus detection was determined on 63 subgingival surfaces and compared with visual findings after tooth extraction. A prevalence of calculus of 22.3% was found on the scanned surfaces, and calculus and cementum were discriminated with a sensitivity of 91% and a specificity of 82%. The positive and negative predictive values were 0.59 and 0.97, respectively. The combined application of the calculus-detection mode and the ultrasonic removal of calculus remain to be investigated12 .
Laser-based technology
The Er:YAG laser has been considered to be the most promising for periodontal therapy. Its ability to ablate soft and hard tissue without major thermal side effects qualifies the use of this laser for periodontal therapy, and Er:YAG lasers at different energy levels have been studied in various in vitro and clinical trials. Er:YAG lasers are solid state lasers that emit pulsed infrared light with a wavelength of 2940 nm, which is strongly absorbed by virtually all biological tissues containing water. The effect of Er:YAG lasers is based on photo ablation. The light-induced tissue evaporation results in water release and a concomitant cooling effect on the surrounding tissue. However, when applied to dental hard tissue, which contains a lower amount of water, increased thermal effects can occur, and therefore water irrigation is required13 .
The only commercially available device (Keylaser3 TM; KaVo) combines detection and treatment in a feedbackcontrolled manner for selective removal of calculus. The integrated calculus-detection device is based on a 655-nm InGaAs diode laser for autofluorescence- based calculus detection (described above as a stand-alone diagnostic tool), whereas a 2940-nm Er:YAG laser is used for treatment. The Er:YAG laser is only activated to emit light if a preselected autofluorescence threshold value for the diagnostic laser on a scale of 0–99 is exceeded. As soon as the value falls below the threshold, the Er:YAG laser turns off. This combination of a diagnostic and a therapeutic laser was designed to optimize calculus removal while minimizing the undesired side effects of the Er:YAG laser14 .
In a clinical study that compared the microbiological and short-term clinical effects after Er:YAG laser debridement vs. ultrasonic treatment. Twenty patients with at least two pockets with a depth of > 5 mm in each jaw were included in the study. The pockets were randomized to receive either feedback-controlled Er:YAG laser treatment (160 mJ per pulse, 10 Hz, chisel-shaped tip of 1.1 0.5 mm, water irrigation) or piezoelectric ultrasonic treatment (Piezon Master 400; EMS). Clinical attachment level gain and pocket depth reduction after 1 month were significantly higher in the laser group, whereas 4 months after retreatment, no significant differences were detected between the two treatment modalities15 .
Another set of clinical trials compared the clinical outcome of periodontal treatment by a feedback controlled Er:YAG laser or ultrasonic instrumentation. Single-rooted and multirooted teeth with pocket depths of > 4 mm were randomly treated in a split-mouth design either by a feedback-controlled Er:YAG laser (160 mJ per pulse, 10 Hz, chisel-shaped tip of 1.65 0.5 mm, calculated energy density 136 mJ per pulse; or chisel-shaped tip of 1.1 0.5 mm, calculated energy density 114 mJ per pulse) or by an ultrasonic device (Cavitron Select; Dentsply, Konstanz, Germany). At baseline, and 3 and 6 months post-treatment, plaque index, bleeding on probing, pocket depth, gingival recession and clinical attachment level were measured at six sites per tooth. Deep pockets showed a tendency to experience more gingival recession, to gain more clinical attachment level and to retain more residual pocket depth compared with moderately deep pockets. Bleeding on probing and clinical attachment level improved significantly in both treatment groups after 6 months compared with baseline. However, statistically significant differences could not be observed between the two types of treatment, suggesting that treatment with the Er:YAG laser was comparable with, but probably not superior to, ultrasonic instrumentation16 .
SUMMARY & CONCLUSION
A number of different technologies have been incorporated into dental devices for the purpose of identifying and selectively removing dental calculus. Some of these new approaches for calculus removal show promising results under optimum in vitro conditions. The Diagnodent designed for calculus detection is not clinically pertinent as only experimental evidence is documented.
An instrument that can integrate calculus detection and removal is highly desirable as it can decrease chair-side time, lead to efficient scaling and avoid overzealous instrumentation. Perioscan which detects and removes calculus simultaneously may be a useful tool in hands of a general dentist with a regular clinical set-up. In comparison to other instruments used for calculus detection and removal Perioscan can prove to be a cost-effective instrument.
Data on the clinical utility of a spectro-optical device for scaling and root planing are scarce. Whether or not a spectro-optical device is useful for calculus detection needs to be evaluated in a clinical setting.
The fiberoptic detection technology shows potential to be a helpful tool in periodontal therapy, but needs to be studied in clinical studies in direct comparison with established scaling techniques.
The laser-based calculus-detection and treatment technology has shown promising results with respect to histology and certain clinical parameters in one study, which, however, was limited to single-rooted teeth. As the total number of cases in the published literature is still small, additional studies are necessary to evaluate the clinical benefit of this technology.
Clinical studies are necessary to assess if the use of these devices can improve long-term treatment outcome, with consequences of smaller residual probing depth, a reduced need for periodontal surgery and less hypersensitivity after treatment.
Supporting File
References
- Van der Weijden GA, Timmerman MF. Asystematic review on the clinical efficacy of subgingival debridement in the treatment of chronic periodontitis. J Clin Periodontol 2002: 29 (Suppl 3): 55–71.
- Biller IR, Kerber PE. Reliability of scaling error detection. J Dent Educ 1980: 44: 206–10.
- Stambaugh RV, Myers G, Ebling W, Beckman B, Stambaugh K. Endoscopic visualization of the submarginal gingiva dental sulcus and tooth root surfaces. J Periodontol 2002:73: 374–82.
- Avradopoulos V, Wilder RS, Clinical and inflammatory evaluation of Perioscopy on patients with chronic periodontitis. J Dent Hyg 2004: 78: 30–8.
- Geisinger ML, Mealey BL, Schoolfield J, Mellonig JT. The effectiveness of subgingival scaling and root planing: an evaluation of therapy with and without the use of the periodontal endoscope. J Periodontol 2007: 78: 22–8.
- Krause F, Braun A, Jepsen S, Frentzen M. Detection of subgingival calculus with a novel LED-based optical probe. J Periodontol 2005: 76: 1202–6.
- Kasaj A, Moschos I, Rohrig B, Willershausen B. The effectiveness of a novel optical probe in subgingival calculus detection. Int J Dent Hyg 2008: 6: 143–7.
- Lussi A, Hibst R, Paulus R. DIAGNOdent: an optical method for caries detection. J Dent Res 2004. 83 Spec No C: C 80–3.
- Krause F, Braun A, Frentzen M. The possibility of detecting subgingival calculus by laser-fluorescence in vitro. Lasers Med Sci 2003: 18: 32–5.
- Folwaczny M, Heym R, Mehl A, Hickel R. Subgingival calculus detection with fluorescence induced by 655 nm InGaAsP diode laser radiation. J Periodontol 2002: 73: 597–601.
- Kocher T, Strackeljan J, Behr D. Feasibility of computer assisted recognition of different dental hard tissues. J Dent Res 2000: 79: 829–34.
- Meissner G, Oehme B, Strackeljan J, Kocher T. Clinical subgingival calculus detection with a smart ultrasonic device: a pilot study. J Clin Periodontol 2008: 35: 126–32.
- Aoki A, Ando Y, Watanabe H, Ishikawa I. In vitro studies on laser scaling of subgingival calculus with an erbium:YAG laser. J Periodontol 1994: 65: 1097–106.
- Konig K, Flemming G, Hibst R. Laser-induced autofluorescence spectroscopy of dental caries. Cell Mol Biol (Noisy-le-grand) 1998: 44: 1293–300.
- Aoki A, Sasaki KM, Watanabe H, Ishikawa I. Lasers in nonsurgical periodontal therapy. Periodontol 2000 2004:36: 59–97.
- Krause F, Braun A, Brede O, Eberhard J, Frentzen M, Jepsen S. Evaluation of selective calculus removal by a fluorescence feedback-controlled Er:YAG laser in vitro. J Clin Periodontol 2007: 34: 66–71.